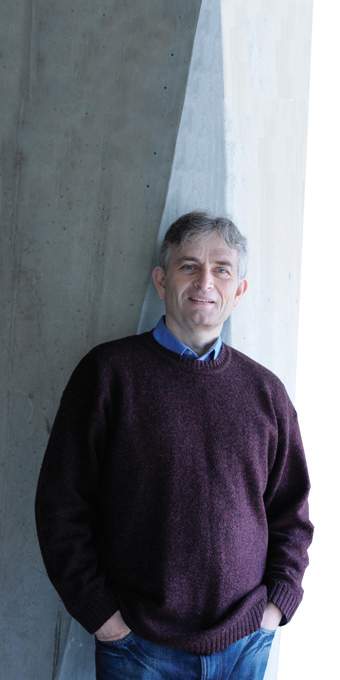
Visitors to the lab of Prof. Eli Zeldov in the Weizmann Institute’s Condensed Matter Physics Department are often treated to the sight of a magnet floating in midair. The trick? Beneath the magnet, a superconductor – a chunk of copper oxide-laced ceramic, cooled to -196°C (-321°F) repels its magnetic field. This feat convinces most visitors that superconductors – materials able to conduct electricity with zero resistance – are super cool.
But it’s not nifty party tricks that make superconductivity such a buzz word these days. The complex interaction between superconducting materials and magnetic fields drives not only the levitation of Zeldov’s modest magnet but also entire trains. From super-fast trains that glide nearly frictionlessly over magnetic tracks at over 600 kilometers per hour to electronic filters associated with cell phone towers to MRI machines, superconductor applications are surprisingly diverse. “Although a lot is known about superconducting materials, there are still many gaps in our understanding as to why and how these substances superconduct in the first place,” says Zeldov.
What scientists do know about superconductors is that magnetic fields can entirely erase the superconductive properties of a material. Superconducting materials, however, often “defend” themselves by repelling external magnetic fields they are exposed to – a phenomenon known as the Meissner effect.
Yet magnetic fields are able to partially penetrate certain types of superconductors; the penetrating magnetic field breaks up into small “islands,” which take the form of tubular whirlpools called vortices. When this happens, superconductivity is maintained outside the vortices, even as it’s destroyed within them. Such vortices pose a problem: They move around through the material in the presence of an electric current and this movement results in resistance. Vortices can thus render a superconductor little better than conventional copper wiring. To impede vortex movement, scientists have devised methods for pinning them to carefully planned defects within the superconducting material, allowing the current to flow more efficiently.
Since the majority of superconductor applications involve magnetic fields, an in-depth understanding of vortex physics has far-reaching practical implications. A team of scientists at Stanford University, including Weizmann alumnus Dr. Ophir Auslaender, who took a leading role in the research, and Zeldov developed an innovative tool that has allowed scientists to get closer to vortices than ever before. In a paper published in Nature Physics, they provided an updated analysis of the microscopic defects at the root of vortex pinning, as well as of vortex structure and dynamics.
The novel method employs a magnetic force microscope; the scientists use its miniscule magnetized tip not only to measure the physical properties of individual vortices, but also to manually move the vortices around in the superconducting material. Thus, the Stanford and Weizmann scientists demonstrated how manipulating a single vortex can be used to probe both the interactions of vortices and the structure of pinning defects within the material. Understanding vortex interactions on such a small scale is invaluable for building the “big picture” of superconductor behavior needed for the effective application of superconductor technology.
The microscope study yielded some surprising results regarding one of the most studied superconducting materials to date – yttrium barium copper oxide (YBCO). This material is among the so-called “high-temperature” superconductors, which lose their resistance at temperatures that, while still inhumanly low, are much higher than the near-absolute-zero temperatures needed for most superconductors. This makes them useful candidates for many applications. Probing single vortices showed that the internal structure of YBCO is much more varied than previously thought, and there were new revelations about vortex dynamics, as well. For instance, by wiggling a vortex while dragging it, the team found that it can be pulled much farther than usual – by an order of magnitude. This finding may help to explain vortex behavior in the presence of alternating currents.
This method has an advantage over most local probing techniques, which only give information about the immediate surface of the superconductor as opposed to what lies beneath. The results of this research have given scientists quite a bit of material for further investigation and modeling is underway to help interpret them. Yet despite the advantages of the technology, this method of measurement is intrusive, as the magnetic tip interacts with vortices in the sample. Zeldov’s team at Weizmann is currently developing a new non-intrusive method for measuring individual vortices – one that will hopefully “levitate” an understanding of superconductivity to a higher plane.
Prof. Eli Zeldov is the incumbent of the David and Inez Myers Professorial Chair.