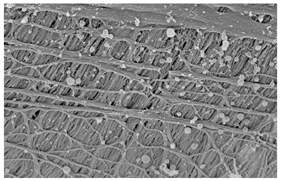
Modern hearing aids are a boon for a great many deaf people, but the quality of the sound they live with is still inferior to that of hearing people. It’s hard to fault the technology – the apparatus we’re born with for translating air vibrations into comprehensible sound is one of nature’s more complex examples of fine engineering. The first step to designing more efficient hearing aids, therefore, is to improve our understanding of the mechanisms that allow most of us to hear such a wide range of sounds, and distinguish among them.
Research by Dr. Itay Rousso of the Weizmann Institute’s Structural Biology Department, which recently appeared in the Proceedings of the National Academy of Sciences (PNAS), suggests that a crucial membrane in the inner ear helps to distinguish among different sound frequencies. This thin structure, called the tectorial membrane, communicates between two structures in the ear: the outer hair cells – which amplify sound in the form of mechanical vibrations – and the inner hair cells – which convert these mechanical vibrations to electrical signals and pass them on to the brain via the auditory nerve. Recent studies have shown that if certain genes for this membrane are missing or damaged, total deafness ensues.
Rousso and research student Rachel Gueta, together with researchers at the Ben-Gurion University of the Negev, wanted to explore the mechanical properties of the tectorial membrane. Using an atomic force microscope, which probes surfaces with a fine microscopic needle, they tested the resistance of the gel-like membrane at various points to assess precisely how rigid or flexible it was. To their surprise, the scientists found that the level of rigidity varies significantly along the length of the membrane: One end of the membrane can be up to ten times more rigid than the other.
These differences occur in the part of the membrane that is in direct contact with the outer hair cells. Observation under a scanning electron microscope revealed that this variation is due to changes in the way the protein fibers are arranged: At one end they form a flimsy, net-like structure that allows the membrane to be flexible; at the other, rigid end the fibers are densely and uniformly packed.
The more rigid the tectorial membrane, the higher the frequency at which it can vibrate. Thus the flexible end of the membrane, which can respond to low frequency vibrations, is close to the hair cells that transmit low frequencies, and the rigid end is near hair cells that transmit high frequencies. This spatial separation, say the scientists, should translate into the ability to distinguish between sounds of different frequencies.
The new understanding of the mechanics of hearing may assist in the development of better hearing aids. Rousso, meanwhile, plans to continue exploring how variations in membrane rigidity affect hearing. The highest frequency our ears can register is a thousand times higher than the lowest, and Rousso intends to test the membrane under different physiological conditions to further understand how it functions, as well as to possibly shed light on the causes of certain hearing problems.
Dr. Itay Rousso’s research is supported by the Clore Center for Biological Physics; the Helen and Martin Kimmel Center for Nanoscale Science; the Jeans-Jacques Brunschwig Fund for the Molecular Genetics of Cancer; the Estelle Funk Foundation; and the President’s Fund for Biomedical Research. Dr. Rousso is the incumbent of the Robert Edward and Roselyn Rich Manson Career Development Chair.