- TAKING ACTION AT STEP 2: TUMOR GROWTH
- Blocking Abnormal Signals
- Encouraging Cell Suicide
- Cutting off Blood Supply
- Early Detection and Diagnosis
TAKING ACTION AT STEP 2: TUMOR GROWTH
Even after a malignant transformation has occurred, the budding tumor is a microscopic lump that doesn’t do much harm until it begins to expand dramatically. This minuscule mass may lurk unnoticed in the body for months or even years (unless it happens to occur in such organs as the skin or cervix, where it can be picked up by vigilant screening). In fact, some tiny growths, called in situ cancer, remain contained indefinitely. They become life-threatening only when they accumulate additional genetic mistakes that allow them to invade adjacent tissues. By identifying the genetic defects that make such explosive growth possible, scientists are seeking to outwit the insidious invader and stop it in its tracks.
Blocking Abnormal Signals
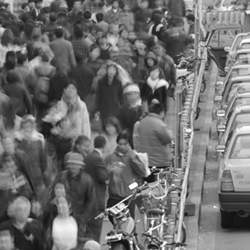
Control over enemy communications - a time-honored military tactic - has become a major weapon in the war on cancer. Communication pathways inside the cell consist of dozens if not hundreds of protein messengers that normally convey myriad signals involved in the growth and maintenance of healthy tissues. But in cancer, the complex and exquisitely orchestrated signaling network is employed to issue just one deadly command: Grow! Weizmann Institute scientists are seeking to interfere with this abnormal growth by intercepting, deciphering and blocking the molecular signals usurped by the tumor-to-be.
Killing the messenger
Like a messenger asked to drop off a package at the reception desk, the signaling molecule that carries the growth command doesn’t usually get inside the cell. Rather, it delivers its message to a receptor on the cell surface, a protruding structure on the outside of the cellular membrane. By binding with the receptor, the signaling molecule triggers a cascade of biochemical processes inside the cell, which relay the signal to the nucleus. In the nucleus, the signal turns on one or more genes that instruct the cell to grow and divide. Normally, receptors serve as a gateway; they allow the passage of growth signals only when they are needed. But in cancer, receptors on the cell surface are stuck in the ON position, continuously dispatching stimulating signals that spur growth. The receptor, therefore, is the first checkpoint where the malignant message can be intercepted, before it even gets a chance to enter the cell.
Prof. Yosef Yarden of the Biological Regulation Department studies a signaling pathway involved in cancers of the breast, ovary, lung, stomach and other organs. He was the first to identify an important growth factor, now called neuregulin, that spurs the growth of malignant tumors. Yarden and his team then solved a riddle concerning the receptors with which neuregulin interacts: one of these receptors, ErbB-2, was long suspected of playing a role in cancer yet it didn’t appear to bind with any known chemical messenger. Yarden clarified that when activated by neuregulin, ErbB-2 teams up with other receptors and acts as an amplifier that reinforces the growth signal received by the cell. The greater the presence of this molecular amplifier on the surface of cells, the more aggressive a tumor is likely to be.
A strategy for “turning off” the molecular amplifier in order to treat cancer is being developed in a collaborative project led by Institute Professor Michael Sela, working in the Immunology Department, and Yarden. The scientists are designing antibodies that would interfere with the transmission of growth-stimulating signals along the neuregulin-ErbB pathway. Apparently, by preventing ErbB-2 from teaming up with growth factor receptors, these antibodies bar the growth signal conveyed by neu-regulin and related growth factors from reaching the cell.
Another Institute team working on neuregulin, headed by Prof. Talila Volk of the Molecular Genetics Department, has discovered that in the cell membrane, much as in real estate, location is key. Using fruit flies as a model, Volk has found that neuregulin works only if present in high concentration in an area of the membrane that comes into contact with other cells. In areas that have no contact with other cells, neuregulin appears to have no effect. The scientists are now trying to clarify the details of this location-specific regulatory mechanism. This work was originally aimed at clarifying the role of neuregulin in the development of the embryo, where this growth factor helps embryonic cells to specialize into different types of tissue. However, the same mechanism also appears to be at work in cancer, and understanding it may help in the design of drugs for blocking growth-stimulating signals before they enter the cell.
A molecular “radar” that makes it possible to track signaling enzymes inside a cell in real time has been developed by the team of Prof. Ben-Zion Shilo of the Molecular Genetics Department. Using the “radar,” the scientists can map the exact progress of certain molecular messengers that play key roles in embryonic development. Shilo focuses on a hormone-like messenger from the neuregulin family called epidermal growth factor, or EGF, and on the specific receptor from the ErbB family to which EGF attaches on the cell membrane. While studying the role of EGF in the development of fruit flies, he has discovered two proteins engaged in a tug-of-war over the EGF receptor: one protein interacts with the receptor to facilitate cell division and growth, while the other prevents further development of certain cells. This research provides a better understanding of how organs develop in an embryo, but it could also lead to the development of cancer drugs that would work by blocking EGF or the receptors for this growth factor.
Inside jobs
Relaying the signal from the receptor on the cell’s membrane to its nucleus is a formidable task: the chemical messengers must cover distances thousands of times greater than their own size and find their way among myriad different substances teeming inside the cell. This task is akin to roaming the crowded streets of an unknown city while trying to deliver a message to a person whose address is unknown. How do the chemical messengers manage to find the nearby relay station under these circumstances? Researchers have found that molecular messengers display specific features that steer them in a particular direction and allow them to recognize the precise molecules with which they should bind in order to relay their signal. By clarifying the details of this delivery system, scientists may learn to block the malignant message before it reaches the nucleus.
Dr. Ari Elson of the Molecular Genetics Department focuses on a relay station just inside the cell membrane. Here certain types of signals are passed along with the help of enzymes called phosphatases, or PTPs, whose job is to remove particular phosphate molecules from proteins. The biological effect of this removal depends on the situation: it results in switching the protein sometimes on and sometimes off. This mechanism constitutes a major link in normal cellular communication, but it can also play a role in cancer development. Elson’s team has already found out that specific PTPs play a role in modulating the amount of phosphate in the proteins of certain types of breast tumors, apparently conveying extra growth control signals to the nucleus. Elson’s ultimate goal is to understand the exact role of PTPs in normal and malignant cells, thereby providing insights for countering malignancy.
Among the major communication routes between the cell membrane and the nucleus are the MAPK cascades - several enzymes that activate one another in succession. Prof. Rony Seger of the Biological Regulation Department focuses primarily on the MAPK cascade known as ERK. It is composed of six sequential steps, some of which set off additional signaling pathways. During normal cell division, ERK turns on for several minutes and then shuts down; in cancer, however, it continues to fire occasionally, leading to excessive cellular proliferation. The end result of the MAPK cascades is the activation of proteins with oncogenic potential in the cell nucleus. Seger's team is aiming to fully understand the role of the MAPK cascades in cancer and how to inhibit them on demand. The scientists have already learned to block various steps in the cascades, and they are checking to see whether the blockage stops cellular growth. They are also studying how hormones and other drugs used to treat cancer affect the MAPK cascades.
Life-saving overdose
All the signals sent to the cell converge in the nucleus, the cellular headquarters that harbors the genes. In response to the signals, some of the genes become activated and issue commands that determine the cell’s fate. When executing growth commands, all living cells call upon chemical assistants called polyamines, which are found everywhere: in the nucleus, in the cytoplasm and also next to the membrane. It is not known how exactly these chemicals assist cellular growth: Do they facilitate the translation of the genetic message in the nucleus? Or do they assist in the synthesis of proteins in the cytoplasm on the basis of this genetic message? Whatever their precise role, the increased presence of polyamines in all vigorously dividing cells, including cancer cells, has turned them into prime suspects in cancerous growth. When polyamines are removed, the cell stops dividing. Therefore, blocking the action of polyamines has become a popular avenue in cancer research.
Prof. Chaim Kahana of the Molecular Genetics Department is exploring several potential strategies aimed at polyamine metabolism. The most obvious approach is simply to inhibit the synthesis of polyamines inside the cell. The problem is, however, that in such cases the cell calls upon extra polyamine assistants from the outside. An alternative approach is to synthesize toxic polyamine look-alikes; these decoys are recruited by the dividing cell, but once absorbed they inhibit cell division and eventually kill the cell. Yet another approach, designed by Kahana’s group, takes advantage of the fact that a cell can tolerate polyamines only up to certain levels; when too many of the chemical “assistants” are present, the cell dies. The scientists, therefore, want to cause polyamines to be selectively overproduced - in cancer cells but not in normal cells; the polyamine “overdose” would then kill the cancer cells.
Encouraging Cell Suicide
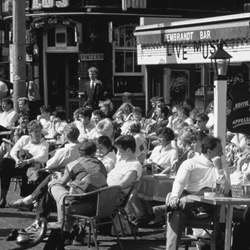
In the short time it has taken you to read this sentence, your body has created thousands of new cells. But there’s no need to worry about the consequences of this booming expansion: it’s part of the normal cell turnover in the body. On an average day, a typical adult creates several billion new cells and kills off just as many. Some cells are destroyed because they are no longer needed. Others are eliminated because they endanger the body; for example, a cell may be killed if it becomes infected by a virus or begins to undergo malignant transformation. Thus individual cells are sacrificed for the good of the organism - just as farmers may decide to eliminate a few infected cattle in order to save the entire herd. The organism has a built-in mechanism for eliminating unwanted cells: every cell contains a suicide program, which can be activated on demand. This preprogrammed self-destruction is called apoptosis, a Greek term that refers to the shedding of leaves. In cancer, however, the suicide program doesn’t work, allowing cells to survive when they should not. Defects in apoptosis also interfere with cancer chemotherapy and radiation therapy because these treatments work largely by activating the cellular self-destruct mechanism. By controlling apoptosis, scientists may be able to induce cellular death for the sake of saving the patient’s life.
Drop-dead gear
One of the most important executioner molecules inducing suicide in unwanted cells is a natural immune system hormone called tumor necrosis factor, or TNF. Prof. David Wallach of the Biological Chemistry Department began studying this molecule more than two decades ago, long before apoptosis became a hot topic in cancer and other areas of biomedical research. His studies have focused on biological processes involving cell death and tissue damage. In the early 1990s, Wallach isolated the receptors to which TNF binds on the cell surface. He then identified a distinct region, or “death domain,” within the TNF receptors that is specifically involved in inducing cell death. In subsequent research, Wallach discovered a key enzyme that serves as the suicide weapon, translating the deadly message into action. This enzyme, called MACH or caspase-8, cuts up certain vital proteins inside the cell, thereby disrupting its normal functions and causing it to die. Wallach’s research has already led to the development of drugs for diseases characterized by excessive cell suicide, such as certain autoimmune diseases. These drugs are being used to treat rheumatoid arthritis and inflammatory bowel diseases, in which they block harmful cellular self-destruction. In cancer, the same research can serve to achieve a different therapeutic goal: to drive malignant cells to suicide by repairing their self-destruct mechanism.
To die for the cause
Programmed cell suicide is particularly important in the ovaries, where thousands of cells in the follicle should die each month, releasing just one mature egg. Failure of these cells to die on schedule causes problems ranging from infertility to cysts or ovarian cancer. Prof. Abraham Amsterdam of the Molecular Cell Biology Department studies the role of cell suicide in both normal and cancerous ovarian cells. He has developed a method to keep granulosa cells, which make up the bulk of the ovarian follicle, alive and multiplying in the test tube, where they can be studied efficiently. Amsterdam’s team has recently discovered that glucocorticoids (hormones such as cortisol and cortisone) and leptin, a substance secreted by fat cells, protect ovarian cells from apoptosis. Both types of substances probably exert their effect through a central behind-the-scenes mediator, the BCL-2 gene, which apparently can suppress apoptosis. “Our primary goal is to learn how to fine-tune ovarian cell death,” says Amsterdam. “The ability to induce apoptosis may lead to future treatments for ovarian cancer.” Amsterdam’s laboratory is also studying the effect of common ovarian cancer drugs, such as cisplatin and gemcitabine HCl, on apoptosis in normal and cancerous ovarian cells, and is developing new methods for the early diagnosis of ovarian cancer.
Recent research in Amsterdam’s laboratory has revealed that theophylline, a widely used asthma drug, makes ovarian and lung cancer cells more vulnerable to such common anticancer medications as cisplatin and gemcitabine HCl. Apparently, theophylline helps induce massive programmed death in the cancer cells. Therefore, by giving theophylline together with common cancer drugs, it may be possible to use the cancer drugs at lower concentrations and so minimize their harmful side effects. Clinical trials in which theophylline is administered to lung cancer patients in combination with cisplatin and gemcitabine HCl are under way at the Tel Aviv Sourasky Medical Center.
Fateful decisions
A suicide-prone cell goes through a stage at which it must make the fateful decision: to live or not to live. This decisional checkpoint is governed by the BCL-2 family of genes, which contains both pro-apoptosis and anti-apoptosis members - genes that can push the hesitant cell toward suicide and those that can persuade it to survive. In malignant cells, the anti-apoptosis members of the BCL-2 family lead to abnormal cell proliferation; they achieve this not by causing cell growth but by preventing apoptosis. Their exact mechanism of action, however, is unknown. Dr. Atan Gross of the Biological Regulation Department has discovered the mechanism that activates one important member of the pro-apoptosis BCL-2 family, a gene called BID, which appears to be a critical molecule in the apoptotic process. When Gross tried to induce life-threatening apoptosis in laboratory animals, normal mice did indeed die from cell loss; in contrast, transgenic mice, which lacked the BID gene, survived. Using both mice and yeast as model systems, Gross’s team is seeking to define the precise role of BID and other members of the BCL-2 family in apoptosis and, by extension, in cancer.
The marrow of a therapy
Research conducted in the laboratory of Prof. Dov Zipori of the Molecular Cell Biology Department may lead to a therapy for multiple myeloma, a malignancy that affects plasma cells in the bone marrow. Plasma cells produce antibodies, which help destroy infectious organisms; but when they proliferate abnormally, they interfere with the production of blood in the bone marrow. In addition, abnormal plasma cells fail to make adequate amounts of antibodies, rendering people suffering from multiple myeloma prone to serious, often life-threatening infections. Moreover, excess plasma cells encroach on bone tissue, causing pain and leading to bone brittleness and destruction. Zipori is studying a molecule called activin A, which triggers apoptotic death in plasma cells. His aim is to clarify the cascade of signals that regulate the birth and death of plasma cells in the blood. Zipori’s team has already managed to decrease multiple myeloma tumors in rats by activating apoptosis in tumor cells.
Cutting off Blood Supply
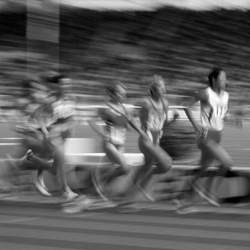
In addition to control over communications, another shrewd war strategy is to deprive the enemy of vital supplies. That is why scientists are looking for ways to deprive a growing cancer of nutrients, growth factors and oxygen. Without a steady supply of these resources, a tumor cannot grow larger than 1 millimeter in size. Even though it may be adding new cells, some of its existing cells will keep dying. Scientists have revealed how the tumor solves the supply problem: It encourages the formation of new blood vessels, which carry the needed provisions to the malignant tissue. Only after this blood supply network is established can the tumor begin to grow explosively. By blocking angiogenesis - the scientific term for the formation of new, hair-thin blood vessels - scientists hope to stop the malignant growth and perhaps even shrink existing tumors. Several drugs blocking angiogenesis are already being tested in clinical trials, while many others may emerge from basic studies being conducted in research laboratories around the world.
Starving the tumor to death
The occasional formation of tiny new blood vessels in the body is a normal, healthy occurrence. For example, it happens every month in menstruation and during wound healing. Normally, angiogenesis is turned off after one or two weeks. In cancer, however, this mechanism is abnormally perpetuated, as are numerous other growth processes. At the Weizmann Institute, scientists are studying the abuse of angiogenesis by cancerous tumors.
While studying blood vessel formation with the help of the magnetic resonance imaging (MRI) techniques that she developed, Prof. Michal Neeman of the Biological Regulation Department was able to explain several puzzling phenomena related to cancer. For example, she provided a molecular explanation for the common recurrence of tumors along the lines of surgical scars: it turned out that surgical incisions trigger a release of substances that stimulate blood vessel formation and tumor growth. She also explained a finding that had long been considered paradoxical: hormones were known to hasten tumor growth, yet when added to tumor tissues in a laboratory dish, they did not induce cell proliferation. Neeman’s research revealed that the hormones cause tumor cells to produce a growth factor that stimulates the production of new blood vessels, which then ferry nutrition to the tumor cells and help them grow. In a laboratory dish, where there are no blood vessels, the rate of tumor growth remains unchanged.
In another project, Neeman discovered that starving tissues of oxygen is an important mechanism that controls blood vessel formation during ovulation, a normal physiological process. In one of her current studies, she is examining the effect of oxygen starvation on the production of new blood vessels in tumors.
Neeman has also revealed intriguing links between angiogenesis and the metastatic spread of cancer. In one study her team discovered that VEGF, the growth factor that acts as the major inducer of blood vessel formation, may stimulate metastasis by increasing the flow in the lymph vessels, which serve as the routes for cancer cell dissemination throughout the body. Another study may explain why ovarian cancer tends to metastasize into the abdomen; the scientists found that LH and FSH, the hormones responsible for regulating angiogenesis in ovarian cancer, prompt malignant ovarian cells to stick to specific molecules found on the peritoneal lining of the abdominal cavity.
Prof. Hadassa Degani of the Biological Regulation Department leads a broad research program aimed at clarifying the mechanisms of breast and prostate cancer, including the role of blood vessel formation in tumor growth and metastasis. In particular, Degani has made major discoveries revealing how blood vessel formation is regulated by hormones. In one landmark study, she clarified the mechanism of action of tamoxifen, the hormonal drug used to prevent and treat breast cancer: tamoxifen starves the tumor to death by destroying its network of blood vessels. More recently, Degani and her colleagues revealed how tamoxifen produces its anti-tumor effects on the molecular level: the drug apparently raises VEGF, the blood vessel-stimulating growth factor, to abnormally high levels. With too much VEGF, the blood vessels become leaky and no longer function properly, depriving the tumor of nutrients and oxygen and leaving it unable to clear waste products. The same study also throws light on the way the female hormone estrogen promotes breast cancer: estrogen, in contrast to tamoxifen, apparently regulates VEGF to just the right levels that meet the needs of the tumor. These findings may lead to a new way of monitoring the effectiveness of hormonal and other therapies based on measuring VEGF levels. Among other projects in Degani’s lab is the study of metabolic processes in malignant transformation, research into the role of glucose metabolism in breast cancer, and development of MRI and molecular imaging methods to monitor the progression and metastasis of breast and prostate tumors.
Early Detection and Diagnosis
Survival is believed to increase when the cancerous tumor is detected early. In fact, the earlier the better; by the time the cancerous tumor reaches 1 centimeter in diameter, about the size of a pea, it already contains up to 1 billion cells, which have the potential of spreading to distant sites and giving rise to additional tumors elsewhere in the body. Weizmann Institute scientists have developed several new methods for improving the detection and diagnosis of cancer in its initial stages.
Tumors get a new look
Magnetic resonance imaging (MRI) is a potentially attractive means of detecting and diagnosing cancer: it is noninvasive and involves no exposure to harmful radiation. However, MRI must first be specially adapted so that it can reveal malignant tumors in a consistent and reliable manner. Major strides in this direction have been made by Prof. Hadassa Degani of the Biological Regulation Department. Her method, called Three Time Point, or 3TP, uses MRI to produce high-resolution images of the tumor and of the surrounding microscopic blood vessels. By revealing the density and permeability of microvessels in tissues, the images make it possible to distinguish between malignant and benign tumors. In clinical trials in the United States, the 3TP method has already been successfully used to diagnose breast tumors in the majority of cases. More recently, Degani and her colleagues have launched a clinical trial in which 3TP is used to diagnose prostate cancer and determine its stage of advancement. Moreover, in an animal study, Degani’s MRI method showed promise in detecting small metastases of breast cancer and evaluating tumor progression. In June of 2003, clearance was obtained from the U.S. Food and Drug Administration for marketing the method in the United States; steps are being taken to provide it for commercial distribution in the European Union and elsewhere.
Electronic finger detects cancer
Prof. Amos Breskin of the Particle Physics Department, working with colleagues from Weizmann and from Soreq Nuclear Research Center, is developing a new tool for the detection and diagnosis of prostate cancer, the most common form of malignancy in men. The technology is being tested in collaboration with urologists from the Kaplan Medical Center. Current screening methods for prostate cancer - digital rectal exam and a test measuring the prostate specific antigen, or PSA, which is elevated in cancer patients - are largely unreliable. For example, the PSA test in many cases sounds a false alarm because PSA levels can be elevated not only in cancer but also in benign diseases of the prostate. Such false positive results lead to unnecessary biopsies, which are fraught with complications. The method developed by Breskin and his colleagues will offer a new, minimally invasive way of examining the prostate gland for the presence of malignant tumors. It consists of an electronic “finger” inserted into the rectum to evaluate the levels of zinc in the prostate. Because prostate cancer causes a drop in zinc, measuring the prostatic zinc concentration and correlating it with the PSA level will make it possible to detect the presence of cancerous tissue with a higher level of confidence. The “finger” makes use of X-ray fluorescence, a technology normally used to detect different elements in materials. Initial results suggest that the number of false positive diagnoses obtained with the new method is three times lower than with the PSA test alone.
Pioneering Steps
From electronics to breast cancer
Prof. Emeritus Ephraim Frei, a founder of electronics research at the Weizmann Institute in the 1950s, spent several decades studying the effects of electromagnetic fields on living tissues. His research led to an innovative breast imaging system that was licensed to the Israeli company TransScan Medical. The technology, called T-Scan, makes use of electrical impedance - a measurement of how electricity travels through a given material - to distinguish between cancerous and healthy cells. In 1999, the U.S. Food and Drug Administration approved a T-Scan device for the detection of breast cancer, citing it as a “significant medical device breakthrough.” Research is under way to apply T-Scan technology to the detection of skin cancers.
Announce your intentions
Neuroblastomas are among the most common solid tumors in young children, but occasionally they transform themselves spontaneously into benign collections of cells. To prescribe optimal treatment, doctors need to know a tumor’s intentions: is it malignant and aggressive or is it turning into a benign growth? Prof. Emeritus Uriel Littauer of the Neurobiology Department has discovered two proteins that may help predict the behavior of neuro-blastomas. Together with colleagues from the Children’s Hospital of Philadelphia, he showed that one protein, MAP2d, is present in large amounts in the cells of malignant neuroblastomas but in far smaller amounts in tumors that have turned benign. Littauer’s Rehovot team then showed that a second protein, 67-kDa LBP, behaves similarly in tumor tissue cultures. Researchers believe that by measuring the amount of these proteins it may be possible to predict the course of a tumor in its early stages.