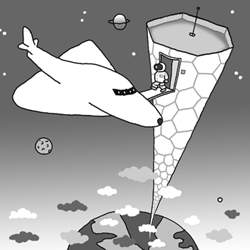
When asked about the practical value of carbon nanotubes, I respond with the same reply given by the great English scientist Michael Faraday to the then Minister of Finance: "One day, Sir, you may tax it." - Sumio Iijima, discoverer of nanotubes
They're incredibly tiny - a millionth of a millimeter in width - and yet are expected to accomplish a multitude of feats. Discovered in 1991, the first nanotubes were made of carbon and captured the attention of scientists worldwide when they proved to be the strongest material ever made as well as being excellent conductors of electricity and heat.
Scientists estimate that a fiber about the width of a human hair made of carbon nanotubes could support around 2 tons. The tubes’ phenomenal strength has triggered research targeting applications ranging from classic engineering quests - such as taller buildings and a potpourri of other structures - to science-fiction-like missions, including cables that would tether a satellite in orbit or even make possible “space elevators” carrying people or equipment into outer space.
Significant challenges remain in carting these tinker tubes from the lab to industry. For starters, their production process results in a random mix of both metallic and semi-conducting tubes. Scientists have yet to figure out how to selectively prepare one or the other, or even how to separate the two types after production.
A nanotube nursery
Tackling one of the hurdles facing the nanotube industry, Dr. Ernesto Joselevich of the Institute’s Materials and Interfaces Department recently chanced upon a possible solution to sort the newly formed tubes according to their potential function: metallic or semiconducting.
“My hypothesis was that I could control nanotube production using an aligning force, such as an electrical field,” says Joselevich. “Just like a compass aligns to the north, the tube’s direction of growth would be controlled by an electrical field, whereas its length would be determined by its rate and duration of growth.”
The approach proved successful, producing nanotubes at a record length of nearly 100 microns. Moreover, Joselevich found that in applying an electrical field to control the direction of growth, he had inadvertently discovered a way of separating the tubes according to function. As long as they were longer than one micron, the tubes were aligned to the electrical field, but once short of this mark, only the metallic tubes were aligned; the semiconducting ones lay in completely random orientations.
Published in Nano Letters, these findings are the first case of a nanotube production strategy that separates metallic from semiconducting tubes as they are formed and may be a launch pad to controlled production. A streamlined production line of this sort would play a critical role in integrating these uncanny tubes into electronic circuits. The method could also be applied to control the growth direction of any material that can be used as a wire, from metals to protein fibers, thus offering potential industrial as well as biomedical applications.
The force
A new study of how carbon nanotubes bind to different materials may advance the development of some of the most effective composites ever.
The study, appearing in Physical Review Letters, was performed by Prof. Daniel Wagner and Dr. Asa Barber of the Institute’s Materials and Interfaces Department, together with Dr. Sidney Cohen of the Chemical Research Support. The team showed that the strength of adhesion between individual carbon nanotubes and an assortment of polymers is between 5-10 times stronger than that between these polymers and the leading composite wonder - carbon fiber.
The principle of forming a composite is to combine two materials to create a new material that is generally far superior to both its individual components.
Researchers first raised the idea of using nanotubes to create stronger composites after studies (including one by Wagner in the late 1990s) showed that they were phenomenally strong - checking in at 100 times stronger than steel and even 30 times stronger than carbon fiber.
But for their idea to succeed, it was necessary to determine that nanotubes would bind effectively to their proposed partners, since a key factor to creating high-performance composites is good adhesion. Only through a successful “merger” could the tubes serve as an efficient reinforcing element to absorb stresses incurred by the material.
Previous studies had attempted to evaluate nanotube adhesion using indirect measures, by examining the adhesion formed between many nanotubes and a polymer and then calculating the average. But these results offered only a limited understanding of the adhesion process. To overcome this problem, the Institute team set out to perform an experimental first - to measure the adhesive force between a single nanotube (where each tube is only a millionth of a millimeter wide) and an embedding polymer. Using an imaging technique known as atomic force micro-scopy (AFM) they first introduced the tube to the polymer and then detached it, since the force required to detach this tube would precisely indicate the force of adhesion.
“This finding raises the hope of creating a new generation of substantially stronger composite materials,” says Wagner. “Potential applications would range from novel aircraft and space technologies to unsurpassed bridges and new nanodevices.”
Breaking the scales
In 1997 Prof. Wagner performed the first experiment aimed at determining the mechanical properties of nanotubes, a new kid on the materials science block.
In measuring how much force could be applied to the tubes before they collapsed, the team’s scales hit 150 gigapascals - thirty times higher than carbon fiber, one of the strongest materials previously known. “Instead of being thrilled I was rather worried,” Wagner recalled with a smile. “How do you publish such extraordinary figures?”
The study, published in Physical Review Letters (1998), was confirmed a year later in computer simulations at NASA and a subsequent experimental study at Northwestern University.
Tubes with a twist
Having set out to study the passage of biological molecules through different membranes, Institute scientists recently came up with something entirely different - a new type of nanotube built of gold, silver and other nanoparticles.
Made at room temperature - a first time achievement, the tubes exhibit unique electrical, optical and other properties, depending on their components, and as such, may form the basis for future nanosensors, catalysts and chemistry-on-a-chip systems.
The study, published in Angewandte Chemie, was performed by Prof. Israel Rubinstein, Dr. Alexander Vaskevich, postdoctoral associate Dr. Michal Lahav and doctoral student Tali Sehayek, all of the Institute’s Department of Materials and Interfaces.
“We were amazed when we discovered the beautifully formed tubes,” says Rubinstein. “The construction of nanotubes out of nanoparticles is unprecedented.
The new nanotube created at the WIS lacks the mechanical strength of carbon nanotubes. Its advantages lie instead in its use of nanoparticles as building blocks, which makes it possible to tailor the tube’s properties for diverse applications. The properties can be altered by choosing different types of nanoparticles or even a mixture, thus creating composite tubes. Moreover, the nanoparticle building blocks can serve as a scaffold for various add-ons, such as metallic, semiconducting or polymeric materials - thus further expanding the available properties.
The resulting tube is porous and has a high surface area, distinct optical properties and electrical conductivity. Collectively, the tubes’ unusual properties may enable the design of new catalysts as well as sensors capable of detecting diverse substances present in minuscule amounts.
A key feature of their success would be the ability, due to the tube’s room-temperature production, to add on biological molecules that would otherwise be destroyed by high production temperatures. These would then perform their natural function of recognizing other molecules in nature, in a key-fits-lock manner. Other tube applications might include lab-on-a-chip systems used in biotechnology, such as DNA chips that detect genetic mutations or evaluate drug performance. Yeda, the Institute's technology transfer arm, has filed a patent application for the tubes.