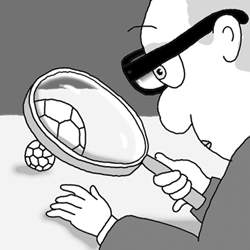
Nanotechnology is the builder’s final frontier - Richard Smalley, co-discoverer of fullerenes
In the early 1980s British chemist Sir Harold Kroto was spending much of his time gazing into space, studying strange-looking carbon molecules found near red giant stars. Thinking that the conditions near these stars might be critical for the molecules’ formation, Kroto decided to contact Rice University scientists, Robert Curl and Richard Smalley, who were studying similar clusters using microwave spectroscopy.
By the end of 1985 the three had made history, discovering a new form of pure carbon containing 60 carbon atoms, which proved to be incredibly stable. While the molecule’s structure was not initially clear, the team suspected that its immense stability could arise only from a spherical shape that remarkably resembles a soccer ball - hence their chosen name for it: Buckminsterfullerene, in honor of R. Buckminster Fuller, an architect with a passion for building geodesic domes.
This newly discovered molecule (popularly known as buckyball), revolutionized the understanding of carbon, giving rise to a new field of chemistry. It earned its discoverers the 1996 Nobel Prize in Chemistry.
Life on the dangling edge
For a while after the discovery of fullerenes, people thought that these super-tough molecules could be formed only by carbon, or perhaps by other carbon-containing materials. But in 1992, researchers at the Weizmann Institute led by Prof. Reshef Tenne discovered an inorganic (carbon-lacking) compound that also forms fullerene-like balls and nanotubes under certain conditions. This finding opened a promising field of research, currently pursued by around 50 groups around the world.
“The key question we asked ourselves,” says Tenne, who heads the Institute’s Materials and Interfaces Department, “is what happens when you scale down a material, bringing at least two of its dimensions (say, length and width) to a scale of roughly 10 - 100 nanometers.” Tenne’s hypothesis was that it would be unstable and immediately fold into the hollow, cage-like structure of fullerenes.
Experimentation proved him right. While preparing thin sheets of tungsten-disulfide for a new kind of solar cells, Tenne’s team noticed that exposing the sheets to high temperatures caused them to acquire strange configurations resembling fullerenes and nanotubes. Subsequent research showed that a multitude of materials shaped in a planar, sheet-like form, behave similarly under the same conditions.
Any material shaped like a sheet of paper is subject to a phenomenon known as the “edge effect,” Tenne explains. The chemical bonds at the edges, called dangling bonds, are unpaired, making them chemically reactive - they seek to pair up with neighboring molecules. This reactivity is too small to have an effect, as long as the material is large enough, but once scaled down to nano dimensions, nearly 50 percent of its molecules are at the edge, causing it to fold.
Slip sliding away
In related research, Prof. Reshef Tenne’s team soon realized that these seamless fullerene-like structures might make an ideal lubricant, a critical requirement of all machinery, from car engines to space ships.
Working with Prof. Lev Rapaport of the Holon Institute of Technology, the team found that inorganic fullerene-like materials made of tungsten disulfide reduced friction in machinery by more than 50 percent, particularly under extreme conditions where conventional lubricants fail. Their study was published in Nature.
The new material was patented by Yeda Research and Development, the Institute’s technology transfer arm, and has recently been transferred from the laboratory to commercial development by NanoMaterials Ltd. Potential applications range from bullet-proof vests and safety-enhanced vehicles to improved hip replacements and machinery lubricants. Even the sporting world might benefit, with precious milliseconds shaved off world records thanks to reduced-friction skis, swimsuits or bikes.
“Basically, everything that moves is subject to friction. This, in a nutshell, explains the importance of effective lubricants,” says Tenne.
According to Tenne, conventional lubricants contain crystallites shaped like flat platelets, with chemically reactive edges. Under working conditions, they stick to machinery parts and undergo chemical reactions that cause them to decompose and rub off. The new molecules, in contrast, are round and inert. They have no dangling bonds at the edges that can catch on to metal surfaces, so they just roll against each other and against the machinery parts, reducing friction between surfaces. They also have an odd, onion-like makeup, which enhances their durability. As the top layers wear off, those underneath maintain a lubricating action. An analysis of the conditions leading to the peeling of layers was undertaken in collaboration with Prof. Sam Safran of the same department.