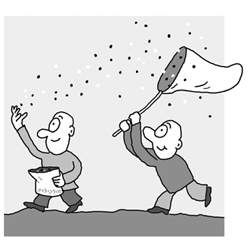
Picture a millimeter, then try divide this into a thousand parts. If you've got a good imagination, you might have just managed to visualize a micrometer. To get to a nanometer divide by a thousand again. If you've managed to visualize that, apply for a job with Steven Spielberg. - A nanometer, as described by NanoInvestorsNews.
New sensors capable of identifying minute traces of various compounds are among the hottest items in nanotechnology. Their applications are in areas ranging from industry and medicine to safety and security. For instance, future “cell-on-a-chip” biosensors might be used to diagnose and monitor diabetes and other diseases, food quality and environmental toxins. Biosensors currently represent a rapidly expanding field with an estimated 60 percent annual growth rate.
Doing what comes naturally
Institute scientists have applied the idea of integrating organic molecules into electronics to create a highly sensitive generic sensor that is based on the unique ability of molecules in nature to “recognize” each other in a key-fits-lock manner.
The sensor, called a molecular-controlled semi-conductor resistor (MOCSER), was developed by Profs. David Cahen of the Material and Interfaces Department, Abraham Shanzer of the Organic Chemistry Department and Ron Naaman of the Chemical Physics Department. Its sensing capacity is determined by the type of molecules glued onto the semiconductor surface.
The molecules are synthesized in such a way that one end binds to the semiconductor surface, while the other end is free to bind to molecules in the target sample. When the molecule bound to the semiconductor “senses” the presence of a specific molecule, it undergoes a change that can be translated by the solid-state device into an electronic signal, providing a clear diagnosis of the sample.
Using a MOCSER, the scientists were able to identify the presence of nitric oxide - a molecule crucial to a variety of biological processes, including brain stimulation. The molecule could be detected down to a minute concentration of a few parts per billion in solutions and in air. Tests with brain tissue were also performed in collaboration with Prof. Menahem Segal of the Neurobiology Department. The device could potentially offer an important early warning system for asthma sufferers.
Tomorrow’s reporters - cells on a chip
Systems of this sort could potentially be used to sense hundreds of substances - from environmental toxins to viruses and food contaminants. At the Institute, Prof. Benjamin Geiger and Dr. Uri Alon of the Molecular Cell Biology Department are addressing some of the obstacles to creating these sensors. Geiger - whose research focuses on the way cells move around the body, adhering to anchorage points along the way - is searching for the best strategy with which to physio-logically connect the cells to the chip. Alon hopes to determine how best to “rig up” the cellular elements destined to serve as fluorescent reporters, on the basis of his experience in tracing the networks of genes and proteins that govern the cell’s response to its environment.
Light reading
Conducting the project were Prof. Yehiam Prior, Dr. Gad Haase and Prof. Ilya Averbukh of the Chemical Physics Department and Prof. Abraham Shanzer of the Organic Chemistry Department.
The team had set out to explore a growing idea in materials science: the use of light instead of electrical current to store and transmit information in electronic devices, thus increasing storage capacity and speed. Their idea was to use light to “read” and “write” information on a nano scale, using a modified form of microscopy.
Current atomic force microscopy (AFM) methods use a sharp-tipped instrument to observe atom-sized features on a surface (see box). But while such methods can see (“read”) the surface topography very well, they cannot alter the surface (“write”) reliably. To address this difficulty, the team set out to study whether the AFM tip could be used to read information by scanning incoming light pulses or similarly, to write information through a series of outgoing light pulses.
The first step, reading information as a sequence of light pulses, proved successful. “We were able to identify molecules on the target surface, even when present in minute amounts,” says Prior. This new diagnostic tool, described in Physical Review, should prove valuable in identifying submicron or nanosized defects in microelectronic devices. Next, the team plans to target the other, more challenging step of writing nanoscale information using light pulses. An achievement of this sort would open a world of possibilities for electronic devices.
Edging in on the atomic scale
In the early 1980s, IBM researchers in Switzerland startled the scientific community with the development of two novel technologies that offered a spectacular view into atomic-scale landscapes: atomic force microscopy (AFM) and scanning tunneling microscopy (STM).
AFM works like an old-fashioned record player, reading surfaces with a needle-fine probe. As the probe moves up and down over this surface it causes a cantilever arm to which it is attached to bend in response. These tiny movements are detected by a laser beam and decoded via a computer, which translates the incoming tactile information into an atom-by-atom image.
STM, which earned its developers the 1986 Nobel prize in physics, works by measuring a quantum effect called tunneling. As it sweeps across the target surface, nanometers away, an ultrasharp probe carrying a small electrical charge causes electrons to “tunnel” across an existing gap. This tiny electrical current is then decoded via a computer to create an atomic-scale image.
Of sieves and sensors
Zeolite minerals have researchers around the world scurrying to uncover their structural secrets. Organized in the form of a porous sieve into which other molecules can fit, zeolites are used in a variety of industrial applications, including petroleum refinement. Nevertheless, researchers believe that these materials might have far more to offer in areas ranging from microelectronics to medical diagnosis - if only it were possible to change a tiny structural detail: the size of their holes.
The potential of these materials stems primarily from their porous and electrically charged nature, which allows them to interact with atoms, ions and molecules throughout their material body rather than at their surface alone. However, the range of chemical interactions that zeolites can promote is limited by the size of their pores. “Zeolite pores are generally 0.5-1.2 nanometers in size, making them inaccessible to many materials, which explains why widespread efforts target the production of larger-pored zeolites,” explains Prof. Daniella Goldfarb of the Institute’s Chemical Physics Department.
Efforts to synthesize large-pore zeolites recently led to the synthesis of a new family of materials (referred to as mesoporous materials) by scientists at Mobil Oil. Goldfarb is currently collaborating with Prof. Shimon Vega of the same department to better understand the surface properties and formation process of these materials. A better understanding of how mesoporous materials are formed might enhance the ability to control their resulting structure and pore size, advancing the production of new catalysts and molecular machines. These would contain porous materials that could function as scaffolds for synthetic and biological materials, serving for instance, as support material for protein-based sensors in medical diagnosis as well as for future nano-wires in microelectronics and optical communications.
Islands of gold
Chemical sensors generally consist of two primary units: a “recognition unit” that detects and identifies substances, and a “reporting unit,” that receives information from the recognition unit and passes it on to a human operator.
A new sensor developed by researchers at the Institute’s Department of Materials and Interfaces allows the construction of flexible reporting units that can successfully communicate with a variety of sensor recognition units. The study was performed by Prof. Israel Rubinstein, Dr. Alexander Vaskevich, then doctoral student Gregory Kalyuzhny and post-doc Dr. Marie Anne Schneeweiss.
The method is based on depositing vaporized gold on a transparent surface (such as glass or plastic), in layers so thin (less than a millionth of a centimeter) they create “gold islands” that are practically see-through. Because of their transparency scientists can beam a ray of light through an island to its other side, where the ray’s intensity and the spectrum of its wavelengths can be measured.
The researchers then bind a recognition unit (such as antibodies that bind to a toxic molecule) to the gold island. Upon recognizing a substance, the recognition units change the way in which the island absorbs the incoming ray of light. This change indicates to the human operator that a substance has been identified, and its specific nature.
The method, described in the Journal of the American Chemical Society, might allow the construction of new recognition units for use in chemical and biological sensors. Yeda, the Institute’s technology transfer arm, has filed a patent application for this approach.
Sensing material defects
Nanotubes may soon join these preventive efforts. Prof. Daniel Wagner of the Institute’s Materials and Interfaces Department has found that the nanotubes (tiny, extremely tough tubes made of a web of carbon atoms) can be used to monitor mechanical stress in materials. His study, published in Applied Physics Letters, revealed that nanotubes offer a highly sensitive means of detecting material defects induced by stress, such as microscopic breaks or holes. Future applications based on this finding may use nanotubes as an early warning system of material fatigue in airplanes and other structures.