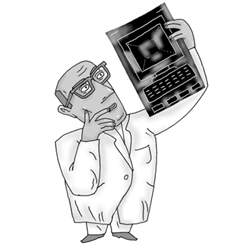
I have discovered something interesting, but I do not know whether or not my observations are correct. - Wilhelm Conrad Roentgen, discoverer of X-rays
In November 1895, German physics professor Wilhelm Conrad Roentgen was in his laboratory studying light phenomena generated by dis-charging an electrical current in a vacuum glass tube when, to his utter disbelief, he suddenly saw the bones of his hand outlined through his flesh.
Roentgen had discovered X-rays. Within weeks, physicians were using these magical rays to see inside the human body and less than three months later, 14-year-old Eddie McCarthy of Massachusetts became the first person to have a broken bone set with their help. The new technology quickly found its way into scientific research, exploding into experimental significance following the 1912 development of X-ray crystallography, which offered a first-time look into the atomic-scale arrangement of crystals. Having exposed crystals to X-ray beams, the father-son team of Henry and Lawrence Bragg, found that the beams diffracted off the crystal’s atoms, and could be captured on film to disclose the crystal structure.
X-ray crystallography has since contributed to the discovery of DNA’s double-helix structure, drug development and far more. Today, sophisticated computational methods are applied to analyzing crystal diffraction patterns.
Crystal clear
Studies of how crystals form may weave together a web of unrelated fields, from those targeting semiconductor technologies, to studies of the origin of life, to the design of polymorphs - crystal formations of key importance in pharmacology.
The common denominator is size. To study these research challenges, Institute scientists apply X-rays to view as well as control the growth of crystals at the atomic level.
Profs. Meir Lahav and Leslie Leiserowitz of the Institute’s Materials and Interfaces Department pioneered the use of grazing incidence X-ray diffraction (GIXD) to analyze the structure of nanosized crystallites formed at the interface between air and water. The investigators are able to work out the exact structure of the crystals formed, according to the way the beam diffracts.
In their analyses, the team has yielded insights into a list of riddles, including how cholesterol crystals form in the body, causing heart disease and gallstones when in excess; the fundamental mechanisms of how water freezes; and the possible routes by which biological molecules such as proteins were first formed. The approach was developed in collaboration with a team of Danish physicists.
The team is currently studying how to control the design and growth of polymorphs - crystals that have different shapes despite being made from the same compound. Polymorphs are of keen interest to the pharmaceutical industry due to their potential influence on drug efficacy. For instance, penicillin crystallized into a form that easily dissolves in the body may be more potent than a penicillin drug packaged in a less soluble crystal. Polymorphs also feature prominently in the production of nanoscopic films used in semiconductors.
Delving into the nanoscopic
Ever tried to find out what’s inside a layered chocolate cake without slicing it? How about tackling a similar task, but at the nanoscale? Take ultra-thin films for instance. Used in diverse applications, from opto-electronics to biological sensors, these films are frequently less than 10-15 nanometers thick. Looking inside these tiny, often multilayered films, calls for highly sensitive probes.
Most existing technologies do not provide information about depth, which is essential for evaluating layered structures. Techniques devised to solve this problem tend to be complicated and frequently damage the sample, distorting the results.
Dr. Hagai Cohen of the Weizmann Institute Chemical Services and Prof. Israel Rubinstein of the Materials and Interfaces Department developed a novel method for evaluating certain ultra-thin films that builds upon a common technique for analyzing material surfaces, known as X-ray photoelectron spectroscopy (XPS).
In XPS, the sample is irradiated with X-rays, causing photoelectrons to be ejected. By measuring the energy level of these photoelectrons, it is possible to determine the atoms from which they originated.
Once the photoelectrons have exited, the remaining sample has a positive surface charge, an outcome researchers traditionally sought to eliminate, since the charging distorts the measurements. However, proving that one person’s stumbling block may be another’s stepping stone, Cohen and Rubinstein realized that the charging effect actually provides structural information - the magnitude of change in charge correlates directly with the atoms’ depth within the film, such that the deeper the atom, the smaller the change. They decided to magnify this effect, causing controlled, easily detectable changes that enabled them to determine both the atom type and its depth within the film.
This Weizmann innovation should yield insights into chemical and biological systems as well as advancing a range of microelectronic applications.