Thousands of physicists from 62 countries will embark in the coming years on one of the most fascinating adventures in the annals of science. Using the world’s largest machine – it weighs in at six times the weight of the Eiffel Tower – they’ll attempt to prove the existence of a tiny, subatomic particle – the Higgs boson. Finding this particle will provide the last piece of a puzzle known as “the standard model” – the most complete and widely accepted theory to date on the structure of all the material in the universe. Failure to find it could shake the world of science like a 9.0 earthquake, necessitating no less than a total rethink of the fundamentals of physics.
The machine is a particle accelerator. It’s nestled in a 27-km-long tunnel, dug some 100 meters beneath the border between France and Switzerland, near Geneva. This accelerator is a part of CERN, the European particle physics laboratory. CERN is a world giant in the field of physics: Its scientists came up with, among other things, the computer languages and protocols that became the basis of the World Wide Web, and it has an effect on the European economy similar to that of the American space program in the U.S. Yet for all its accomplishments, the scientists at CERN, with all their complex machinery, haven’t managed to track down this one missing particle. Their best hope yet lies with the new accelerator being built, called the Large Hadron Collider (LHC), which will be able to accelerate bundles of protons to 99.999998% of the speed of light. These bundles will be aimed straight at each other, causing collisions that will release so much energy, the protons themselves will explode. For less than the blink of an eye, conditions similar to those that existed in the universe in the first fraction of a second after the big bang will be present in the accelerator.
At that first moment, the universe was simple, hot and very energetic. As the seconds ticked by, space expanded, and that energy began to dissipate. The universe cooled, becoming more complex as it did so, until it reached the level of complexity we know today. Inside the collider, the scientists will try to recreate those simpler, primordial conditions – a cosmos in which all particles were simply “different faces” of a small number of elementary particles, and the four fundamental forces that act between those particles were but expressions of a single force. As a first step in reconstructing that primal force, scientists have managed to join two of those forces: the electromagnetic force and the weak nuclear force. (The other two are the strong nuclear force and gravity.) But the existence of this “electro-weak” force presupposes the existence of a particle called a “Higgs” – named after the Scottish physicist Peter Higgs who, along with Robert Brout and Francois Englert, first predicted it. The only fly in the ointment is that since its prediction over 40 years ago, no Higgs particle has yet been detected.
A number of Weizmann Institute physicists have joined in the effort to find the missing Higgs particle. They’re a somewhat multigenerational scientific family – Prof. Giora Mikenberg, who heads the Israeli team, is the teacher and mentor of Prof. Ehud Duchovni, who taught Prof. Eilam Gross. Also working with these three are Dr. Vladimir Smakhtin, Dr. Daniel Lellouch and Dr. Lorne Levinson, all of the Particle Physics Department and the Nella and Leon Benoziyo Center for High Energy Physics.
High-Speed Collisions
Inside the accelerator, powerful, head-on collisions take place continuously between the protons, resulting in highly energetic particles that wink in and out of existence in a tiny fraction of a second. To obtain proof of their existence, one must identify the traces they leave behind. Thus a number of particle detectors have been created, each designed to trap a different kind of particle. The Weizmann team led by Mikenberg has developed a special detector, constructed at the Institute and other places around the world, which will contribute to detecting the elusive Higgs. “Elusive” may be an understatement: The chances of being able to find a Higgs particle in a single collision are about the same as those of coming up with a specific cell from a specific leaf on a specific plant by plucking one cell at random from all of the plants on the whole planet.
The LHC, equipped with superconducting magnets that work at temperatures of less than 2 degrees above absolute zero (absolute zero is -273° C), will produce something like a billion collisions per second. If protons were people, the collision rate would entail every person on the planet running into every other person on the planet every six seconds. Calculating and analyzing the data from all of these collisions will be akin to listening in on all of the planet’s telephone conversations at once, assuming the entire population is talking simultaneously on 20 phones apiece.
Hidden Dimensions and Black Holes
In addition to the Higgs particle, the LHC might, at some time in the future, produce millions of very tiny black holes. This surprising idea arises indirectly from string theory, which posits that the particles we know are simply manifestations of one “fundamental constituent,” called a string, and all the forces acting in nature are nothing more than different aspects of one single primeval force. Reality, as suggested by this theory, contains at least eleven dimensions, but seven of them are “curled up” and shrunk so small they can’t be observed.
Another recent model has suggested that the gravitational force can propagate in the additional dimensions, and that the size of the curvature of some of these additional dimensions might not be so small. Under these assumptions gravitational force becomes very strong at short distances, in particular for very energetic (massive) particles. Close to particle collision sites, this can lead to an enormous concentration of gravitational power in a small area. If this happens, a black hole might form. In fact, if calculations are correct, black holes could be created in the LHC at a rate of up to one per second. There’s no need to worry though: The physics of black holes dictates that the smaller the black hole, the higher its temperature. Such tiny black holes will be so hot they’ll vaporize almost as soon as they come into existence.
Prof. Giora Mikenberg’s research is supported by the Nella and Leon Benoziyo Center for High Energy Physics. Prof. Mikenberg is the incumbent of the Lady Davis Professorial Chair of Experimental Physics.
Prof. Ehud Duchovni’s research is supported by the Nella and Leon Benoziyo Center for High Energy Physics.
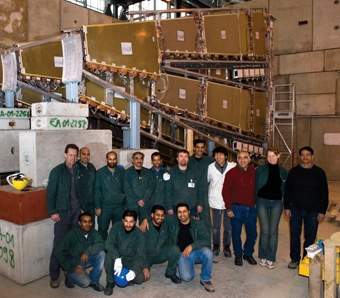
Prof. Ehud Duchovni
Prof. Ehud Duchovni was born in Israel in 1953. In his youth he was an Israeli swimming and target-shooting champion. He served in an elite army unit and was later a target of a terrorist attack. He was awarded a medal for bravery by the Israeli Police, and the Verdienstkreuz am Band by the German President, for his actions in this attack. Later, while on reserve duty, he was wounded in the back. Duchovni is married to Noga and is the father of Inbal, Eynat, Gilead and Avner.
Prof. Giora Mikenberg
He was born Jorge Mikenberg in Buenos Aires, Argentina, in 1947. When he was just 16, he left his family in South America, changed his name to Giora, and set out for Israel to live on a kibbutz. In Israel, with encouragement from Prof. Yehuda Shadmi, he began to study physics, eventually ending up at the Weizmann Institute of Science. In the army, he served under Sergeant Ehud Duchovni, who would become his pupil. At CERN, Giora is known as George.
Prof. Eilam Gross
Prof. Eilam Gross was born in Tel Aviv. After completing his army service in an elite communications unit, he left for New York to study music. There he came across a cult book, The Tao of Physics, which prompted him to come back to Israel and study physics at the Hebrew University of Jerusalem. His master’s thesis at the Weizmann Institute was written on string theory, after which he “deserted” theortical work for experimental high-energy physics and the team of Prof. Mikenberg. Today, between mathematical formulas and charting particle trajectories, he continues to work on his music, and he dreams of staging a performance that will combine music with insights gained from particle physics. He is the father of two daughters, Nuphar (20) and Yaara (15).