It's war. And it's not just "out there,"but inside our bodies, where a fleet of immune agents is constantly on the lookout for rogue invaders - viruses, bacteria, and fungi. Over the past few decades, as scientists have tirelessly traced the micro-trenches of our body's battle zones, scoring increasingly promising victories in the form of antibiotics, vaccines, and even the anti-AIDS-virus cocktail, hopes have risen that one day we may also succeed intargeting foes from within, such as cancerous tumors.
"One of the most compelling questions is why the body is unable to mount an effective attack against its aberrant cells," says veteran immunologist Prof. Gideon Berke of the Weizmann Institute of Science. "The puzzle is that the immune system actually starts by generating a potentially promising response - namely, killer cells that can seek out and destroy a tumor. Yet within a short time these cells all but disappear while the tumor marches on."
Berke specializes in white blood cells known as cytolytic T lymphocytes (CTLs), ingenious killer cells present in nearly every organ. Lining his office walls are volumes of publications dating back to the late 1960s, when he was a graduate student working with Haim Ginzburg. They were the first to discover the in vitro generation of killer cells and to analyze specific details of their killing activity. The 70s, 80s, and early 90s found him and scores of scientists worldwide striving to determine the T-cell's molecular mode of action in greater detail.
These efforts led to the surprising, and perhaps frustrating, finding that in parallel to the T-cells'attack on them, cancer cells are able to mount a counterattack. Furthermore, they use the very same weapon employed by T-cells - a mechanism first proposed by Berke and others back in the early 90s (see box). The key is a lethal interaction between a molecule called FasL, present on the attacking cell, and a death receptor on the target cell named Fas. Upon activation the Fas receptor triggers a built-in cell suicide mechanism known asapoptosis.
The discovery that tumor cells, like T-cells, also use FasL in self-defense stirred immense excitement. "It was surprising, clever, elegant,"says Berke. But then conflicting evidence started to emerge, including the finding of tumors that did not express FasL and the fact that there was often no difference between the fates of patients expressing FasL and those who did not.
T-cell fratricide
Berke and his colleagues have now shed light on this puzzle using in vivo-induced killer cells. In a study recently published in Immunology, the team, including graduate student Jie-Hui Li and Dr. Dalia Rosen, together with Prof. Paul Sondel of the University of Wisconsin, here on sabbatical at the time, confirmed that both tumors and killer T-cells are endowed with FasL and Fas receptors. Each "camp"is able to kill the other, yet tumors seem to have the upper hand. They can also cause T-cells to turn on one another, killing other cancer-fighting T-cells as well as innocent bystanders (see diagram).
These insights into cell death induced by tumor/immune skirmishes are already influencing clinical oncology. One new approach aims atdetermining a patient's prognosis or optimal treatment based on the presence or absence of FasL and Fas in cancerous cells. In related research, Berke is developing a test to determine tumor sensitivity to existing cancer drugs, relying on afluorescent dye that labels cells undergoing cell death following exposure to a drug. The test has so far proven successful in gauging the susceptibility of breast and colon cancer tissue to different drugs.
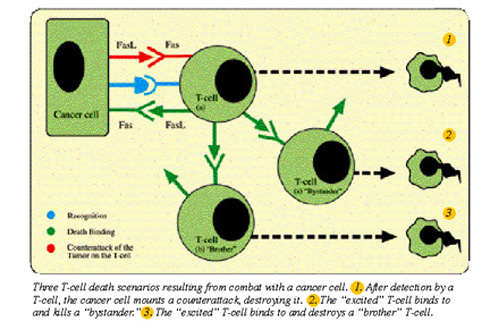
Raining winning ideas
Looking out the window of his bus en route to a meeting in Cambridge, many years ago, Berke was suddenly struck with an idea that would later become a leading thread in his scientific thinking. "It was a rainy September day,"he recalls, "and the fields were a gloomy yellow."Having grown up in Israel, Berke was used to dust-colored summer fields hungry for rain, but it seemed highly curious in England, where it was raining cats and dogs. "The idea suddenly came to me that there must be a genetic programming that makes plants die even when they have sufficient nutrients and water,"says Berke. "They die because they are supposed to."
In 1991, this idea of an in-built cell-death mechanism led Berke to propose a new theory of how killer T-cells target their foes, challenging the then-prevalent hypothesis championed by Pierre Henkart of the U.S. National Institutes of Health. Henkart had suggested that T-cells kill by releasing substances that perforate the target cell membrane. These perforations then enable certain enzymes to penetrate the target cell, causing DNA fragmentation and apoptosis.
The trouble was that Berke and electron microscopy expert Dr. Dalia Rosen, working with certain killer cells, couldn't find any evidence supporting either the presence of these enzymes or the formation of membrane lesions. Concluding that an alternative pathway must exist, they proposed that killer cells function by activating built-in receptors in the target cell, which in turn trigger the cell's demise. This theory later proved right on the mark when researchers in France and Japan discovered the cell's FasL / Fas apparatus.
Tumor tactics
Masters of deceit, cancer cells employ a variety of schemes to outwit the immune system. These include:
· Counterattack: The tumor fights back, suppressing the immune response.
· Camouflage: Variants of the tumor are created, lacking the features that would mark them for destruction.
· Sidestepping: The tumor deflects the immune attack by producing anti-apoptotic proteins that neutralize the immune cells'weaponry.
Prof. Berke holds the Isaac and Elsa Bourla Chair in Cancer Research.
Garlic “Smart Bomb”
Weizmann Institute scientists have destroyed malignant tumors in mice using a chemical that occurs naturally in garlic. The key to the scientists’ success lies in the development of a two-step system that delivers the cancer-treating chemical directly to the tumor.
The active chemical, called allicin, is the substance that gives garlic its distinctive aroma and flavor. For many years, scientists studying allicin have known that it is as toxic as it is pungent. It has been shown to kill not only cancer cells, but the cells of disease-causing microbes and even healthy human body cells. Fortunately for our cells, allicin is highly unstable and breaks down quickly once ingested. However, its rapid breakdown and undiscriminating toxicity have presented twin hurdles to creating an effective allicin-based therapy.
Researchers in the Institute’s Biological Chemistry Department have now solved this double challenge, designing a delivery method that works with the pinpoint accuracy of a smart bomb. The study, reported in Molecular Cancer Therapeutics, was performed by Drs. Aharon Rabinkov, Talia Miron and Marina Mironchick, working with Profs. David Mirelman and Meir Wilchek.
Allicin is not present in unbroken cloves of garlic; it is produced in a biochemical reaction between two substances stored apart in tiny adjoining compartments within each clove - an enzyme called alliinase and a normally inert chemical called alliin. When the clove is damaged - whether by food-seeking soil parasites or upon crushing in cooking - the membranes separating the compartments are ruptured, alliin and alliinase interact, and allicin is produced.
The scientists wondered whether it might be possible to reproduce this natural reaction at the site of a tumor. In this way, toxic allicin molecules could be aimed directly at the cancer cells.
To pursue this goal, the scientists took advantage of the fact that most types of cancer cells exhibit distinctive receptors on their surfaces. They took an antibody naturally programmed to recognize one of these receptors and chemically bonded it to the garlic enzyme, alliinase. They then injected this paired unit into the bloodstream, where, as expected, it proceeded to seek out cancerous cells. The second component, alliin, was then injected at intervals. When encountering the alliinase enzyme, the normally inert alliin molecules were turned into lethal allicin molecules, which then penetrated and killed the tumor cells. Neighboring healthy cells remained intact due to the precise delivery system.
Using this method, the team has succeeded in blocking the growth of gastric tumors in mice. The tumor-inhibiting effects were seen up to the end of the experimental period, long after the internally produced allicin was spent. The scientists note that the method could work for most types of cancer, as long as a specific antibody could be customized to recognize receptors unique to the particular cancer cell. The technique could prove invaluable for preventing metastasis following surgery. “Even though doctors cannot detect where metastatic cells have migrated and lodged themselves,” says Mirelman, “the antibody-alliinase unit should be able to hunt them down and, in the presence of alliin, destroy them anywhere in the body.”
From vampires to fungi
Long hailed as a wonder plant, garlic has been used traditionally for everything from warding off vampires to treating bacterial and fungal infections. The ancient Egyptians fed garlic to their pyramid-building slaves to enhance their stamina; the Greeks used it to treat bladder infections, leprosy and asthma; and in World War I, following research by Louis Pasteur proving garlic’s anti-bacterial properties, the plant was used to disinfect open wounds and prevent gangrene.
Modern research has confirmed that garlic has antibacterial, antiviral, antifungal, anti-amoebic, anti-inflammatory and even heart-protecting properties, with a lipid-lowering effect that prevents the accumulation of fatty deposits. Allicin, the active compound in crushed garlic, has been shown to kill over 20 types of bacteria, including Salmonella and Staphylococcus.
In previous research, Prof. Mirelman and his colleagues Dr. Miron, Dr. Rabinkov and Prof. Wilchek revealed that allicin kills disease-causing amoebas, bacteria and fungi by inactivating some of their enzymes. This finding led to current efforts to develop therapies against intestinal and fungal diseases, which claim the lives of thousands yearly and afflict millions more. The group has also shown that allicin can function as an antioxidant, inactivating harmful oxygen molecules believed to contribute to atherosclerosis.