01-09-2004
TAKING ACTION AT STEP 3: METASTASIS
To win the war on cancer, scientists must overcome the most deadly aspect of this disease - its ability to invade normal tissues and spread to distant organs. A surgeon can effectively remove a single tumor, but when cancer extensively invades healthy tissues and disseminates secondary tumors throughout the body, surgery can no longer cure the disease. In fact, its spread throughout the body, called metastasis, is precisely what makes cancer deadly. Fewer than 10 percent of cancer deaths are caused by tumors growing at their original site; in the majority of cases the metastases are the real killers. Scientists at the Weizmann Institute are striving both to clarify the molecular mechanisms involved in metastasis, which is still one of the least understood aspects of cancer, and to devise strategies against the lethal malignant invasion.
Preventing Cell Migration
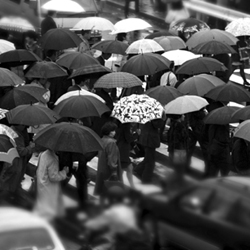
Apart from white blood cells, which regularly make the rounds of different tissues, most body cells are homebodies: they stay put in the same tissue for life; if they do float away, they die. Cancer cells, unfortunately, are different; they aggressively invade adjacent tissues and are capable of traveling through the bloodstream, surviving the journey, and giving rise to metastases in new places. Scientists are trying to keep cancers from spreading by foiling the ability of malignant cells to move about.
Respect thy neighbor
Normal cells are generally good neighbors; they speak to one another regularly, heed their environment and even limit their offspring to prevent overcrowding. Communication among them takes place when they adhere to their surrounding substance, the matrix, and to one another. This adhesion anchors cells in one place and enables them to link up to form tissues and organs and to exchange signals that control their behavior. In contrast, tumor cells are bullies that ruin the neighborhood, and they are known to be associated with poor adhesiveness.
Prof. Benjamin Geiger of the Molecular Cell Biology Department studies the molecular mechanisms underlying cell adhesion and the signals resulting from adhesive interactions. By understanding these mechanisms and signals, scientists hope to clarify how defects in adhesion enable cancer cells to multiply uncontrollably. The scientists also hope to shed light on metastasis: the adhesive properties of cancer cells have a major effect on the capacity of these cells to detach from their neighbors, penetrate into adjacent blood vessels and migrate to distant organs, where they form metastatic tumors. Using advanced light and electron microscopy, Geiger and his colleagues have shown that cell adhesion sites exhibit extraordinary structural and molecular diversity. Geiger’s team has also discovered a link between adhesion signals and the so-called cell cycle clock, a crucial molecular apparatus that governs cell division and is believed to be disrupted in virtually all types of human cancer. Other studies in Geiger’s laboratory examine the involvement of adhesion and motility in metastasis and develop new approaches for blocking the metastatic spread.
Prof. Alexander Bershadsky of the Molecular Cell Biology Department is exploring the interplay between cell adhesion and the system of internal cellular structures known as the cytoskeleton. He has revealed how the cascade of chemical signals triggered by the adhesion molecule p120 catenin affects a variety of proteins in the cytoskeleton. The findings have explained the mechanism by which the mobility of cells is reduced when they come into contact with one another. This mechanism plays an important role during tissue formation, but in invasive cancerous cells it is often impaired. Bershadsky and his colleagues have also discovered that the points of contact between cells and the extracellular matrix act as mechanical sensors that inform the cells about the physical characteristics of their microenvironment. This “sensory” mechanism is also impaired in cancer cells, which usually cannot tell the difference between rigid and soft surfaces.
The physical forces that determine a cell’s fate are the object of several collaborative studies led by Geiger and Bershadsky. In one study, conducted by a multidisciplinary team of Institute physicists, chemists and biologists, scientists applied microelectronics technologies to biological processes to develop a method for measuring the minuscule physical forces involved in cell adhesion. This research has revealed how these forces affect the cascade of biochemical signals transmitted into the cell, which, in turn, alters cell fate and behavior.
If detached from the surrounding matrix, cells usually die within a short time, a phenomenon called anoikis (the Greek for homelessness). What enables “homeless” cancer cells, detached from the original tumor, to survive the journey to a new site? Prof. Yehiel Zick of the Molecular Cell Biology Department has cloned a previously unknown gene, called galectin-8, which is apparently responsible for the survival of migrating prostate cancer cells. This gene appears to be involved in regulating normal cell adhesion, but it is unusually active in advanced prostate cancer. Why does the prostate tumor step up its galectin-8 activity? What kinds of advantages does the gene confer on the cancerous cells? Zick seeks to answer these questions and hopes to design inhibiting molecules that would block galectin-8, stripping prostate cancer cells of their lethal ability to roam the body's tissues.
Good migrations
Every minute, thousands of white blood cells, the immune system’s “soldiers,” leave the circulatory vessels to patrol body tissues, looking for signs of infection or injury. When embarking on their journey, they know how to respond to special “password” molecules displayed on the blood vessel wall. Unfortunately, this beneficial migration system is exploited by cancerous cells, which also travel through the circulatory system and penetrate body tissues, causing metastases in distant organs. The cancer cells are also able to respond to the “password” molecules and gain access to neighboring tissues. Identifying these signaling molecules and understanding how they function may therefore yield a powerful therapeutic tool. Prof. Ronen Alon of the Immunology Department has revealed that immune cell migration is controlled by molecules called chemokines, which display themselves on the vessel wall to allow specific cells to pass through. He found that chemokines also mark the exit route, guiding the migrating cells until they leave the vessel. Recent findings suggest that certain types of tumor cells can also respond to particular chemokines and may therefore be guided by chemokine signals to the same sites as migrating immune cells. In addition, Alon’s team clarified the role of blood flow in the cellular crossing of the blood vessel wall. These new insights into cell migration from the circulatory system may contribute to future therapies that will fine-tune cell migration from the blood to the surrounding organs - enhancing beneficial immune responses or blocking undesirable migration by cancerous cells.
Dr. Idit Shachar of the Immunology Department has discovered a molecular mechanism that exerts powerful control over the migration of two types of white blood cells, T and B cells. Shachar found that the adhesion of T and B cells to the extracellular matrix, a vital step in their journey through body tissues, can be prevented by the protein called interferon-gamma. Shachar’s team is currently exploring the potential of interferon-gamma, which suppresses excessive inflammation, to serve in addition as a drug against metastatic lymphoma: in both disorders, T and B cells have an increased capacity to enter various organs, and by blocking this capacity it may be possible to relieve inflammation or to prevent the spread of metastases. In a related project, Shachar studies adhesion genes that play a role in chronic lymphocytic leukemia, which is characterized by the abnormal proliferation of B cells. By evaluating the activity of the adhesion genes, it may be possible to make a precise diagnosis and determine the stage of this type of leukemia - perhaps one day to develop a treatment that would offset the abnormal adhesion.
Killing the Tumor
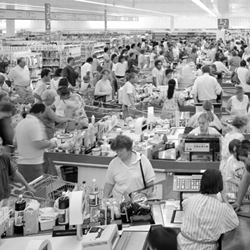
When a tumor can be surgically removed, the operation usually solves the problem. Difficulties arise when surgery is impossible, as for example, when metastatic tumors spring up in many places. In such cases, the standard treatments are chemotherapy or radiation. But in addition to affecting the tumor these therapies destroy healthy tissues, causing severe side effects. Weizmann Institute scientists are working on several approaches for destroying cancerous tissue in a precise, targeted manner. Such methods promise to render treatment more effective and reduce side effects to a minimum.
A green solution
Photodynamic therapy, recently approved in the United States for the treatment of advanced esophageal, bladder and lung cancers and for age-related macular degeneration, involves injecting a patient with special light-sensitive drugs, or photosensitizers. These drugs are harmless, but they become toxic when exposed to light. When the treatment site is illuminated, the photosensitizer becomes locally activated and generates toxic materials, particularly reactive oxygen molecules that kill living cells; at the same time, the damage to surrounding healthy tissues, which are not exposed to light, remains minimal. However, photosensitizers presently in clinical use have several serious drawbacks that limit their utilization in photodynamic therapy. Prof. Avigdor Scherz of the Plant Sciences Department and Prof. Yoram Salomon of the Biological Regulation Department have developed a “green” photosensitizer, one based on chlorophyll, the same pigment that makes green plants and photosynthetic bacteria such effective light collectors. The “green” drug offers crucial advantages over previously used photosensitizers, including an improved ability to penetrate bulky tumors and a faster clearance from the patient’s body. The Weizmann technique involves administering the pigment molecules into the blood and then using fiberoptic surgical techniques to locally illuminate the tumor; the blood vessels feeding the tumor are destroyed within minutes, and as a result - following a single treatment - the tumor tissue dies and disappears. Scherz and Salomon’s method is presently being tested in clinical trials of prostate cancer at two medical centers in Canada, and more trials with this type of cancer are expected to begin in Israel. Applications for the treatment of other types of cancer are in the pipeline. The technique could provide a powerful new weapon in the war on cancer.
Tumors smell the danger
A potential new cancer therapy developed in the Institute’s Biological Chemistry Department hails back to an ancient natural remedy, garlic, long believed to contain substances with antitumor properties. Modern studies show that allicin, garlic’s main biologically active ingredient and the one responsible for its smell, does indeed kill cancerous cells in a laboratory dish.
However, until recently pure allicin could not be used as a therapy because it is highly unstable. Moreover, a way had to be found to deliver allicin to cancerous cells without killing healthy tissues. Weizmann researchers - Dr. Aharon Rabinkov, Dr. Talia Miron, Dr. Marina Mironchik, Prof. David Mirelman and Prof. Meir Wilchek - have solved both these problems by designing an ingenious method that mimics the way allicin is formed in nature. This substance, which protects the garlic plant from soil parasites and fungi, is generated when garlic cloves come under assault, as for example when a clove is crushed or invaded by a parasite.
The crushing brings together two garlic components, the enzyme alliinase and the inert substrate alliin, which are normally separately stored; their mixing produces the smelly guard, allicin. In the Weizmann Institute approach, scientists have chemically bound the enzyme alliinase to monoclonal antibodies that recognize tumor markers on cancer cells. The resultant alliinase-antibody conjugate is injected into the patient’s circulation and lodges in tumor tissue but not elsewhere. Several hours later the scientists inject the second component, the inert alliin, which interacts with the alliinase enzyme now attached to the tumor cells and triggers the formation of allicin, which in turn kills tumor cells without affecting the healthy neighboring cells not outfitted with alliinase.
In preliminary experiments on mice, the method prevented tumor development after cancerous tissue was implanted into the mouse. If confirmed in further studies, the approach could be particularly useful in the treatment of metastatic cancer by blocking the development of secondary tumors.
An attractive therapy
Unnecessary damage to healthy tissues caused by cancer drug therapy could be avoided if the drugs were dispatched directly to the tumor in guided-missile fashion. Such targeted delivery is being developed by Prof. Ruth Arnon of the Immunology Department. Arnon is taking advantage of a biological “superglue” developed by Profs. Meir Wilchek and Edward Bayer of the Weizmann Institute’s Biological Chemistry Department. The “superglue” is based on the strongest biological attraction known to exist in nature, that between a vitamin called biotin and an egg-white protein called avidin. Arnon and her colleagues started out by targeting biotin to tumor tissue via tumor-specific antibodies. They then attached avidin or its bacterial relative, streptavidin, to chemotherapeutic drugs. As expected, the attraction of avidin and streptavidin to biotin drew the drugs to the tumor, where they destroyed the cancer. In an alternative approach, the scientists targeted modified streptavidin to specific tissues or organs, subsequently introducing biotin-drug complexes, which homed in on these tissues or organs. Using this method, scientists have, for example, caused the common cancer drugs 5-fluorouridine and cisplatin to accumulate in the liver in high concentrations over a prolonged period. The approach may lead to an efficient new way of targeting tumors in various organs with appropriate drugs.
The hormone connection
Steroid sex hormones are known to stimulate the growth of certain types of malignant tumors, particularly those of the reproductive system. Thus about 30 percent of breast cancers are dependent on the sex hormone estrogen, while approximately 70 percent of prostate cancers are testosterone-dependent. A common therapy for these cancers makes use of drugs that mimic the action of gonadotropin-releasing hormone (GnRH), which regulates the sex hormones. However, one of the problems with these drugs, called GnRH analogs, is that they quickly disappear from the bloodstream. Prof. Mati Fridkin and Dr. Shai Rahimipour of the Organic Chemistry Department and Prof. Yitzhak Koch of the Neurobiology Department have designed and synthesized new GnRH analogs that solve this problem. The compounds developed at Weizmann are so sturdy and stable that they remain in the blood for many hours without being broken down; this prolonged activity promises to render them vastly more potent than existing medications.
In a separate set of experiments, the scientists bound their GnRH analogs to a light-sensitive drug used in cancer treatment called photodynamic therapy (see p. 43). The intention behind pairing the two substances is twofold. First, it renders the therapy more targeted: since many tumors have GnRH receptors, the combined molecule selectively zeroes in on the tumor while sparing healthy tissue. Second, the combined molecule is expected to deliver a double blow to the tumor because each of its two components produces a separate anti-cancer effect.
Immune Strategies
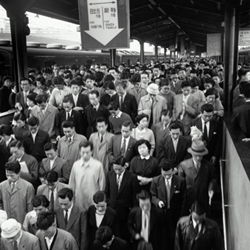
Wouldn’t it be ideal if patients could become immune to cancer? The notion that the immune system, which defends the body against various diseases, may also offer protection against cancer existed already in the 19th century. Physicians noticed that tumors sometimes shrank in cancer patients who contracted bacterial infections, and they hypothesized that the immune system, along with fighting the infection, also attacked the tumor. Several researchers tried to develop immune strategies for fighting cancer in the 19th and 20th centuries, but until some two decades ago their ideas were not broadly accepted because they failed to produce consistent results. Recently, however, thanks to enormous advances in immunology, interest in immunotherapy of cancer has resurged. Today scientists are striving to understand how tumors evade the immune attack and how to make the cancer more vulnerable to the immune system’s weapons. The ultimate goal is a focused approach: Rather than boosting the immune system in general, scientists are seeking to selectively mobilize specific immune mechanisms capable of defeating cancer.
A winning combination
Since completing his Ph.D. at the Weizmann Institute in the early 1970s, Prof. Zelig Eshhar of the Immunology Department has been studying molecular recognition in the immune system - the mechanisms by which immune cells and molecules “recognize” each other. This research has led to the development of a cancer therapy that combines the advantages of two immune system components: antibodies, which are capable of recognizing tumors but cannot efficiently penetrate them, and white blood cells called T cells, which in principle can carve through cancerous tissue but often lack the ability to recognize and home in on the tumor. Eshhar produced hybrids he nicknamed T-bodies - T cells equipped with the recognition ability of antibodies. Human T-bodies have completely eliminated prostate and breast tumors in laboratory animals. After proving the feasibility of T-body technology in the lab, Eshhar spent a sabbatical year at the U.S. National Cancer Institute, where he worked with immunotherapy pioneer Dr. Steven Rosenberg to bring the approach closer to clinical application. Today Eshhar's approach has been picked up by more than two dozen research teams around the world that are designing T-bodies for treating cancer, autoimmune diseases and infection with HIV, the virus that causes AIDS. The safety of the approach is already being tested in cancer patients at three medical centers in the United States, including a trial at the National Cancer Institute targeting advanced ovarian cancer. Efficacy trials are being planned, several of them using Eshhar's T-bodies.
Cancer vaccine
Cancer vaccines work on the same principle as vaccines against flu or other infectious diseases; their goal is to prompt the immune system to generate specific and effective weapons for protecting the body against the disease. But whereas flu vaccines are aimed at preventing disease, most cancer vaccines are aimed at treating, rather than preventing, malignancy. In cancer, the vaccine’s job is to stimulate the immune system to destroy tumor cells. Earlier studies in laboratories around the world had shown that by injecting laboratory animals with genetically modified tumor cells, it’s possible to galvanize the immune system’s killer T cells into combating the tumor. In particular, an important study conducted by Prof. Emeritus Michael Feldman and Prof. Lea Eisenbach of the Immunology Department, showed that this approach worked against late-stage, metastatic cancer. The study also revealed that tumor cells contain certain proteins that are different in quality and quantity from those in normal cells, and that killer T cells can be specifically activated to recognize these differences.
Eisenbach went on to provide the first evidence that a much simpler approach could be just as effective: rather than immunizing mice with tumor cells, she used a vaccine composed of short protein fragments, or peptides, derived from cancer cells. In cancer cells, these peptides may be mutated in a unique way or be present at abnormally high levels compared with normal cells. Therefore, by vaccinating animals with such peptides, scientists can prompt the immune system to recognize and attack tumor cells while sparing normal tissue. When mice with advanced lung cancer were injected with Eisenbach’s vaccine, their immune systems launched a powerful T cell attack that reduced the number of existing tumors and prevented metastasis. Eisenbach has developed a unique model for identifying the peptides and proteins that can activate killer T cells against human tumors: she makes use of mice whose immune systems have been partially replaced with those of humans. She and her colleagues have already identified candidate peptides and proteins for vaccines against various types of advanced cancer, including metastatic cancers of the breast, colon, prostate and bladder. A trial in human patients with advanced breast, ovarian and lung cancers is expected to be launched at a major Israeli hospital.
Killer schemes
Why doesn’t the immune system mount an effective and sustained attack against malignant cells? This question is explored in the laboratory of Prof. Gideon Berke of the Immunology Department. As a graduate student at Weizmann more than 30 years ago, Berke and a colleague were the first to discover several basic properties of the immune system’s killer T cells. Since then, Berke has focused on clarifying the role of killer T cells in protecting the body against viruses and tumors. In the early 1990s, challenging an accepted hypothesis, he showed that the molecular mechanism by which the T cells deliver their lethal blow is not as straightforward as was then believed; rather, the cells can perform the killing using two different mechanisms. In later research he suggested that tumors could become invisible to the immune system by evading one of the T killer mechanisms. Berke is currently exploring the details of the mechanism used by tumors to shun immune system surveillance. In a separate project he is developing a test for determining tumor sensitivity to existing cancer drugs. The test relies on the use of a fluorescent die to label cells undergoing programmed cell death, or apoptosis. Changes in fluorescence reflect the rate of cell death in a tumor sample exposed to a given cancer drug and could predict whether the drug is likely to be effective in killing the tumor.
Fighting Advanced Cancer
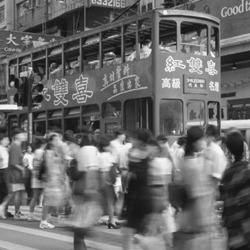
Even after cancer has progressed to an advanced stage or metastasized to distant organs, all hope need not be lost. Weizmann Institute scientists are working to improve existing treatments for advanced cancer or to devise new approaches for treating the most stubborn, seemingly intractable malignancies.
A therapy to the bone
Bone marrow transplantation grants many patients with leukemia a new lease on life. In leukemia, the bone marrow is diseased and no longer produces the correct amounts of blood components. To treat the disease, the patient’s bone marrow is destroyed by strong radiation and high-dose chemotherapy and replaced with a transplant of healthy marrow from a donor. The procedure depends on finding a compatible donor; yet sadly many patients fail to find a suitable donor among family members or even among the millions of volunteers in international registries.
Prof. Yair Reisner of the Immunology Department has pioneered a method that makes it possible to perform mismatched, or incompatible, transplants. This approach, developed in collaboration with Italian physicians, is based on the use of megadoses of bone marrow. While in matched transplants the patient and the donor must share all immunological markers, the megadose approach makes it possible to perform a transplant even if the patient and donor share only half of the markers. Virtually all patients have a family member who can serve as such a donor, making transplantation available for the vast majority of candidates. After being proved successful in leukemia patients at Italy's Perugia University, the megadose approach is now used at several dozen hospitals in Europe, the United States, Israel and elsewhere. Reisner and his colleagues are now working to reduce the amount of “conditioning” - the preparatory steps performed prior to bone marrow transplantation. A milder conditioning regimen - for example, one involving lower radiation doses - can minimize the risks associated with the treatment. This research may lead to “gentler” bone marrow transplantation for the treatment of patients with a relatively long life expectancy, including people with various malignancies and autoimmune diseases.
Bone marrow contains primitive, primordial cells called stem cells, which mature into different components of blood. In some cases, curative transplants for cancer patients are made up of stem cells alone, rather than of the entire bone marrow. Prof. Tsvee Lapidot of the Immunology Department studies the development and migration of human stem cells, both normal and leukemic. His team has discovered key elements of the mechanism responsible for the migration of human stem cells from circulating blood into the bone marrow. Furthermore, the scientists have managed to greatly increase the proportion of human stem cells capable of migrating to the marrow, thereby improving transplantation success in immune-deficient mice. In another project, Lapidot revealed new differences between normal and leukemic stem cells that could be used for selectively purging out leukemic cells prior to transplantation. These and other studies in Lapidot’s lab may lead to improved stem cell transplantation.
Mother Nature to the rescue
Natural molecules that protect the body against disease are finding their way into the treatment of advanced cancer. Prof. Michel Revel of the Molecular Genetics Department has played a leading role in the discovery and study of two natural molecules now employed as drugs. In the late 1970s, Revel isolated the gene for interferon-beta, a human protein that fights viral infection in the body and is used as a drug against a variety of ills, including certain types of cancer - particularly glioma and non-small-cell lung carcinoma.
Another important molecule isolated in Revel's lab is interleukin-6, or IL-6, an immune system protein involved in defending the body against infection and inflammation. Because this protein boosts the production of blood platelets, it can offset the loss of blood cells that often accompanies intensive forms of traditional cancer therapies - chemotherapy or radiation. IL-6 may also improve blood cell formation after bone marrow transplantation.
Moreover, IL-6 can play a role in cancer treatment itself. One possibility, now being tested in clinical trials, is to use IL-6 to improve the effectiveness of vaccines against advanced cancer such as melanoma. Studies conducted by Revel with the Weizmann Institute’s Prof. Lea Eisenbach revealed that IL-6 prevents the development of metastases in animals, probably through the same immune mechanisms as those at work in vaccines. In another promising research direction, Revel’s team created an IL-6 “chimera” - a recombinant molecule that boosts IL-6’s therapeutic potential. In animal tissue, the “chimera,” consisting of IL-6 and its receptor fused together, has been shown to block a protein important for the survival of melanoma cells. The Weizmann scientists have started collaborating with Italian researchers to investigate the effects of the IL-6 “chimera” on human melanoma tumors. In addition, in collaborative research with the Institute’s Prof. Tsvee Lapidot, the IL-6 “chimera” molecule was found to improve the success of blood stem cell transplantation.
Interferons - natural anti-viral proteins - have been approved as drugs for treating viral diseases and various types of cancer. However, their use is limited by undesirable side effects; moreover, cells sometimes develop resistance to interferons or release antibodies to neutralize the drugs. These limitations may be overcome if scientists achieve a molecular understanding of how interferons bind to their cellular receptor. The laboratory of Prof. Jacob Anglister of the Structural Biology Department is aiming to elucidate the three-dimensional structure of the outer part of the interferon receptor, the part that protrudes beyond the cell membrane. The scientists are also studying the complex formed by the receptor with one particular interferon - interferon-alpha2. This research is conducted using nuclear magnetic resonance spectroscopy, a powerful tool for studying the three-dimensional structure of proteins. The structural information gained from this study is expected to pave the way for the design of interferons and interferon-like molecules with greater therapeutic results and fewer harmful side effects.
Using different technologies Prof. Gideon Schreiber of the Biological Chemistry Department studies the complexes formed by interferons and their receptors. Schreiber focuses on an important puzzle in the field: how is it that interferons alpha and beta bind to the same receptors on the cell membrane but produce different effects inside the cell, turning on different genes at varying intensities. Because standard methodologies have failed to produce an adequate image of the complexes' structure, Schreiber’s team developed a new strategy. Their approach is to experimentally identify points of “docking” between the two proteins and incorporate these points into a so-called docking algorithm, a computer program that creates a three-dimensional image of the complex. The docking points are identified using a sophisticated method, double mutant cycling, which systematically introduces mutations into the amino acids making up the protein in order to study this protein in great detail. Understanding the details of the complex interactions between different interferons and their receptors promises to provide means for designing improved interferons to fight disease.
Achilles’ heel of drug resistance
Chemotherapy, used in millions of cancer patients, often fails to save lives because cancer cells become resistant to multiple chemotherapeutic drugs. Dozens of laboratories around the world are therefore seeking to circumvent cancer drug resistance. However, no treatment aimed specifically at resistant cells is yet available. Prof. Moti Liscovitch of the Biological Regulation Department has discovered what he believes is an Achilles’ heel of multidrug resistant cells; he hopes that this discovery will lead to new drugs for the treatment of multidrug resistant cancer.
Cancer cells, unlike normal ones, can usually survive and grow without receiving signals from their support structure, the extracellular matrix; in fact, their independence from the matrix is a major factor contributing to their unruly growth. But Liscovitch found, rather surprisingly, that multidrug resistant cells regain a degree of “normality”: they undergo changes in their membranes that apparently restore their need for a signal from the matrix. His lab is currently striving to identify the precise nature of this signal and to clarify the route by which it gets into the cells. The need for the signal is precisely the vulnerable point of resistant cancer cells; Liscovitch hopes that by depriving these cells of the external signal he can interfere with their growth and even their survival. The approach promises to lead to a therapy that would block the growth of multidrug resistant cancer cells in a precise and targeted manner, just like the arrow that struck the mythical Greek warrior in the heel.
Data Mining Speeds Up the for Cancer Genes
SYSTEMS BIOLOGY
Thanks to modern gene research technologies, any scientist in the world can now tap into the 3 billion genetic “letters” of the recently deciphered human genome. Using DNA chips, scientists can simultaneously measure the activity of 20,000 genes. But faced with this overwhelming “haystack” of information, how does one find the “needle” - the gene or genes involved in a particular biological process such as cancer?
Prof. Eytan Domany of the Physics of Complex Systems Department has developed one of the first and most successful data processing tools that help scientists make sense of the genetic information glut. In more than a dozen projects performed in collaboration with other researchers at Weizmann, as well as with scientists and physicians from other institutions in Israel and abroad, he is applying this method to answer critical questions in numerous cancer studies, such as: What is the genetic signature of a particular cancer? And how can one tell from its genes whether a tumor is going to spread aggressively?
Domany's approach is a sophisticated data mining method that uses concepts from physics, mathematics and statistics to find patterns and hidden subtle relationships in heaps of data. To understand the challenge, imagine being in a large stadium filled with football fans. The vast majority of them are screaming at random, but about a dozen are singing a particular song in unison. How do you pick out the tune from the noise produced by thousands of screamers? The general idea is to identify the singing fans, put them in one stand and point a microphone at them, so that you can listen only to their song and not to the background racket. Much in the same manner, Domany's method helps scientists scan thousands of genes with the help of DNA chips and zero in on the handful that work together to perform a particular task in cancer.
Examples of projects in which the method is applied to cancer include: searching for genes that trigger certain types of leukemia; identifying breast cancer patients who need more aggressive therapy; clarifying the genetic characteristics of breast and prostate cancers that produce metastases after a short time; establishing whether some cases of childhood leukemia may have viral origins; and determining whether primary and recurrent brain tumors that look the same in a biopsy have similar genetic features. Some of the projects involve collaboration with numerous hospitals and universities around the world. For example, in a large-scale study funded by the U.S. National Institutes of Health, Domany is collaborating with physicians and scientists from Cornell University, University of Medicine and Dentistry of New Jersey, Memorial Sloan-Kettering Cancer Center, Princeton University, Rockefeller University and the Institute for Advanced Study to define the genetic profile of colon cancer in its different stages.
These studies promise to speed up the advent of personalized medicine, in which cancer therapy will no longer be a one-size-fits-all affair; rather, in each case, the treatment will be tailored to the patient's genetic profile and to the genes involved in that particular type of cancer.