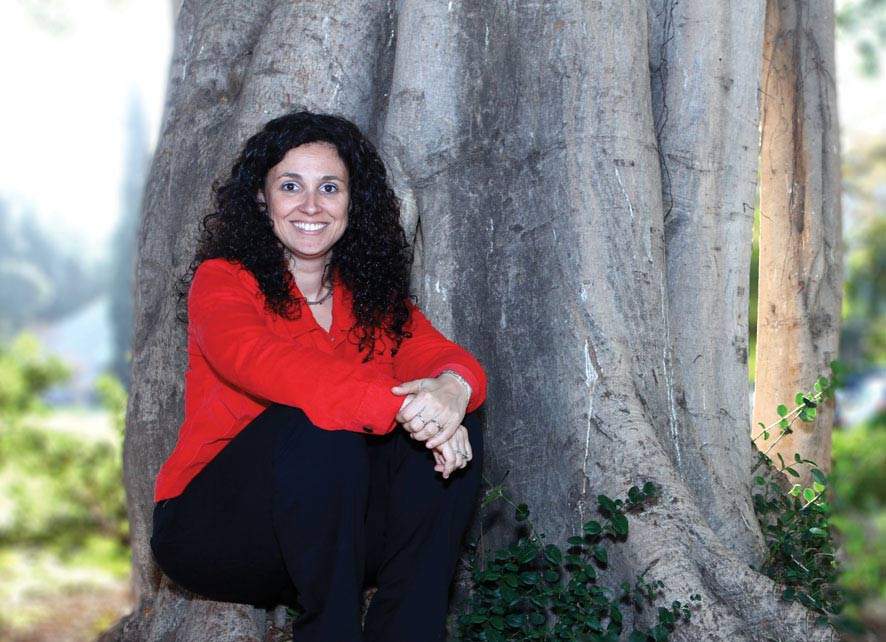
We all care about having healthy levels of cholesterol and other fats in our blood, but our blood vessels themselves don’t seem to care. Even when plaques of fat threaten to block the flow of blood, the vessels fail to respond to the danger, when they might, for instance, sprout new branches. Conventional wisdom has it that the vessels don’t “know” the fat is there. But don’t they? And wouldn’t it be wonderful if they did sense the danger and branched out to bypass the blockage, thus preventing heart attacks?
Exploring the relationship between blood vessels and fat is one of the two major projects in the lab of
Dr. Karina Yaniv, who recently joined the Biological Regulation Department as a senior scientist. She addresses this question as part of a more general theme: clarifying how blood vessels form in the embryo.
The cells that form blood vessels, called endothelial cells, have long been known to be sensitive to blood oxygen levels: If these levels drop, new vessels grow in the vicinity to ensure the surrounding tissue gets an adequate oxygen supply. As for fat, until now scientists believed that the vessels didn’t “feel” its presence. Yet during her postdoctoral studies at the National Institutes of Health in the United States, Yaniv provided the first experimental evidence for a new hypothesis according to which endothelial cells are attuned to the fat availability in their environment and respond accordingly. Using zebrafish as a model, her research revealed that when fat supply is low, developing zebrafish embryos grow extra blood vessels, probably to ensure adequate levels of vital nutrients. After all, the embryo is nourished mainly by fat molecules, such as those in egg yolk. When fat is plentiful, vessel growth in the embryo comes to a halt.
In adulthood, this biochemical dialogue between fat and blood vessel walls could be misfiring: High cholesterol levels in the blood might be preventing collateral vessels from growing, even though such growth is precisely what’s needed to prevent blood vessel blockage. In her new lab at the Weizmann Institute, Yaniv seeks to identify the molecular signals exchanged between fats and the endothelial cells of the blood vessel walls.
Understanding how blood vessel growth is regulated could have important implications for human health, possibly even enabling the regulation of such growth on demand. Helping the body grow new vasculature could be beneficial after a heart attack or stroke, or in preventing these events. Conversely, in cancer, selectively blocking the formation of new blood vessels that feed the tumor could help in treating malignancy.
Zebrafish Vessel Formation in vivo
This movie shows timelapse multiphoton confocal images of vessel dynamics in fli-EGFP transgenic zebrafish. Elongating angiogenic sprouts migrate between the somites to give rise to a dorsal branch. Highly dynamic behavior involving extension and retraction of numerous filopodia can be seen as the sprouts extend dorsally.
Zebrafish do have a lymphatic system
When not studying the development of blood vessels in the embryo, Yaniv focuses on the lymphatic system, which is essential for the immune response, fluid metabolism and fat absorption. During her postdoctoral research, she showed that, contrary to common belief, zebrafish do have a lymphatic system. Thanks to her research, several labs in the United States and Europe have started using zebrafish in their studies of the lymphatic system. Yaniv also provided the first in vivo evidence in support of a century-old hypothesis concerning the origin of the lymphatic system: Her studies showed that in the vertebrate embryo, the lymph vessels originate in the veins. Because the lymphatic system is involved in numerous disease processes, this research, too, could help in the treatment of disease. In particular, metastatic cells use the lymphatic network to migrate to distant organs; knowing in greater detail how lymph vessels form during embryonic development might make it possible to block their growth around a malignant tumor, preventing the spread of metastasis.
Yaniv has chosen to conduct her studies on zebrafish because they offer an excellent model for her genetic research. Their small size allows her to keep a staggering 12,000 fish in 400 one-foot-high aquaria in an average-size lab. In the transparent embryos, blood and lymph vessels are easily visualized. Moreover, their genes are convenient to manipulate: Though all the fish swimming merrily in Yaniv’s aquaria look identical, sporting the same “zebra” stripes, some are transgenic, their genomes containing “reporter” genes that emit a glow marking particular DNA segments, while others are mutant, missing certain genes. The range of mutants prepared by Yaniv’s lab promises to help reveal numerous genetic secrets vital for human health.
Development of the Lymphatic Vascular System in Zebrafish
This movie shows two-photon time-lapse images of transgenic zebrafish expressing GFP in the nuclei of endothelial cells. Two lymphatic endothelial progenitors and their daughters have been highlighted in yellow and red for ease of tracking. Endothelial nuclei in the midline of the embryo migrate rostrally and then ventrally. Just ventral to the dorsal aorta they divide and the daughter cells incorporate into the wall of the newly formed thoracic duct - the main lymphatic vessel of the fish.
Creative time management
Born in Cordoba, Argentina, Dr. Karina Yaniv immigrated to Israel in 1989 as part of a Zionist youth movement program. After a year on Kibbutz Magal, she enrolled in the Hebrew University of Jerusalem, earning a Ph.D. in developmental biology from Hadassah Medical School in 2005. She then conducted postdoctoral research at the National Institutes of Health in Bethesda, Maryland, and joined the Weizmann Institute faculty in the summer of 2009. Yaniv is married to Ram, a software engineer, and is a mother of three: Yotam, 12, Nitay, 9 and Eylon, 4. She says combining motherhood with scientific research takes creative time management, such as taking a break from work in the afternoon and resuming it late at night. Working hard is part of her nature: “If you do something you love, you want to do it well,” she says.
Dr. Karina Yaniv's research is supported by the Willner Family Center for Vascular Biology; the Carolito Stiftung; the Abraham and Sonia Rochlin Foundation; Lois Rosen, Los Angeles, CA; the estate of David Arthur Barton; the estate of Paul Ourieff; and the estate of George Talis.
Unraveling Batten Disease