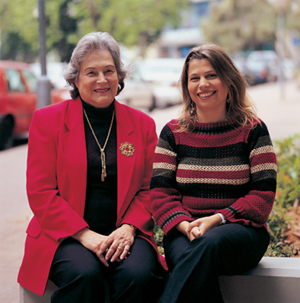
Viruses are exceptionally efficient creatures. They’re not even really “alive” in one sense, lacking a reproductive mechanism of their own. And yet they multiply so effectively - at the expense of their host cells - that they often kill in the process.
It’s a sneaky business, based on a key-fits-locks mechanism. The virus first anchors onto its host, whereupon a protein in its envelope attaches to a fitting receptor on the cell membrane. Having gained access to the cell’s headquarters - its nucleus - the virus incorporates its genetic material into the host’s DNA machinery, causing it to produce hordes of the invading viral particles.
Scientists working to block viral diseases are developing vaccines aimed at preventing this initial anchoring. The accepted approach involves introducing a weakened or killed virus, which the immune system learns to recognize, generating defensive antibodies. Future encounters with a live virus will trigger a swift immune response.
But viruses don’t just sit back and take it. In a rapid evolutionary process, they frequently change their surface protein “keys,” thus evading discovery - it’s similar to an ongoing struggle between code makers and code breakers.
The flu virus, for example, changes the shape of its envelope proteins almost annually - making effective vaccines nearly useless the following year. But Prof. Ruth Arnon and Dr. Tamar Ben-Yedidia of the Institute’s Immunology Department might now change this.
The scientists focused on one of the surface proteins of the flu virus, called hemagglutinin, which consists of three molecular chains wrapped around one another like rose petals. When the virus anchors itself on a living cell, these “petals” open to reveal a previously hidden peptide, which - the scientists found to their surprise - is unvaried across a range of viral strains. In other words, while hemagglutinin frequently changes its external petal-structured envelope to avoid detection, the hidden peptide has remained conserved throughout evolution. BINGO! - a potential Achilles’ heel has been uncovered. By targeting this fixed “weak link” of the virus, the scientists reasoned, it might be possible to develop a vaccine that would deliver a long-lasting - rather than merely annual - blow.
And lab results suggest that they are right on the mark. Working with (then graduate student) Dr. Rafi Levy, Arnon introduced a molecule containing one of the hidden peptides into mice. The result: 50 percent of the vaccinated mice showed resistance to infection by a range of flu viral strains. The results were even more telling when the mice were treated with vaccines containing three different “hidden” peptides: 90 percent of them demonstrated immunity. Moreover, even seven months later, 50 percent remained protected.
Encouraged by these findings, Arnon and Ben-Yedidia started examining the immune response of mice implanted with a human immune system. They used a new vaccine consisting of four different “hidden” peptides intended to provoke an immune response in humans. Though subsequently infected with a very large, usually fatal, dose of the virus, all of the mice survived.
Following these successful studies in mice, BiondVax, a recently established start-up, now plans to continue the development of this approach and perform clinical trials in humans. The goal is to design a five-year nose-drop vaccine that would be effective for people of all ages. “It’s an entirely different concept from existing vaccines,” Arnon says. “The nose-drop vaccine - of which only a single drop will be needed - should effectively target a range of flu virus strains.”
 Flu virus. Constant renewal%3B (right) Hemagglutinin protein. Hidden peptide in red%3B dark areas indicate frequently changing regions.jpg)
Not the common cold
The flu (or influenza) virus is different from the common cold. It attacks the respiratory tract (nose, throat and lungs) and usually comes on suddenly. Resulting symptoms include a fever, headache, congestion and muscle aches.
Most flu strains are mild, meaning that they have at least a few familiar features that our immune system recognizes and is able to fight - generally involving a recovery period of one to two weeks. Yet even with these mild flu strains, some people develop life-threatening complications, such as pneumonia - indeed, influenza annually claims the lives of roughly 36,000 people in the United States alone. Those at higher risk include toddlers, the elderly and people with chronic medical conditions, such as diabetes, asthma and heart disease.
Rarely, extremely virulent flu strains crop up that are so different in their genetic make-up our immune system is completely helpless - as in the pandemic of 1918, which killed between 20 and 40 million people around the world. One story tells of four ladies who were healthy enough to play bridge together into the night. Three of them were dead by the morning.
Prof. Arnon’s research is supported by the Y. Leon Benoziyo Institute for Molecular Medicine; the Milton A. and Charlotte R. Kramer Foundation; Mr. and Mrs. Eugene Applebaum, Bloomfield Hills, MI; Lester and Evelyn Burton, Bingham Farms, MI; Dr. and Mrs. Claude Oster, Bloomfield Hills, MI and Dr. and Mrs. Irving Slott, Chicago, IL. She is the incumbent of the Paul Ehrlich Professorial Chair of Immunology.
Opening the Window
Prof. Yair Reisner's research is supported by the J & R Center for Scientific Research; the Belle S. and Irving E. Meller Center for the Biology of Aging; the Gabrielle Rich Center for Transplantation Biology Research; the Abisch Frenkel Foundation for the Promotion of Life Sciences; the Loreen Arbus Foundation; the Crown Endowment Fund for Immunological Research; the Mario Negri Institute for Pharmacological Research - Weizmann Institute of Science Exchange Program; the Charles and David Wolfson Charitable Trust; Renee Companez, Australia; Mr. and Mrs. Irwin Goldberg, Las Vegas, NV; and Mr. and Mrs. Barry Reznik, Brooklyn, NY. Prof. Reisner is the incumbent of the Henry H. Drake Professorial Chair in Immunology.