 with graduate students Mark Eisenberg and Tali Kobilo. Learning to forget.jpg)
While some people try to remember, others try unsuccessfully to forget. People whose memories have a debilitating effect, such as trauma sufferers who remain haunted by their experience throughout their lives, would benefit from the ability to intentionally dim or erase specific memories without affecting the others.
A step toward reaching this goal has recently been made by a Weizmann team. The scientists have uncovered a fundamental rule governing the workings of the brain. The findings were recently published in Science.
Every memory that we acquire undergoes a "ripening" process (called "consolidation"), which lasts a few hours after the memory is formed. During this time, certain treatments can erase that memory. Until recently, the accepted belief was that every memory consolidates just once, and after that the window of opportunity for erasing that memory closes.
However, recent evidence has shown that calling up a memory might again make it susceptible to disruption. If true, it might be possible to activate a "memory eraser" immediately after the act of remembering, even though years may have passed since the original memory was formed.
Yet leading labs in the world, spurred on by that evidence, came up with seemingly contradictory results: Working with animals, they found that only in some cases did it appear possible to erase old memories upon recall.
The current findings, made by Prof. Yadin Dudai of the Neurobiology Department, explain the puzzling discrepancies in the previous findings and shed light on how memories are recalled and stabilized.
Winner loses all
To understand the findings, think of the bits of information stored in our memories. Each may have many associations, some conflicting with others. For instance, a certain food can arouse memories of taste - delicious or disagreeable; a person can be remembered in pleasant or unpleasant contexts, and so on. When we next taste the food or see the person, all of the associated memories are evoked. But in the end, only one of them will determine our reaction (i.e., become dominant). This memory dictates whether we will eat the food or reject it, whether we will smile at our acquaintance or ignore him. Dudai's team, which included Mark Eisenberg, Tali Kobilo and Diego Berman, found that only the memory that won the competition for dominance became sensitive to erasure. It is this memory that must be consolidated once again before being reinstalled in long-term memory. In other words, the winner, under the appropriate circumstances, loses all. In Dudai's words, "The stability of the recalled memory is inversely correlated with its dominance."
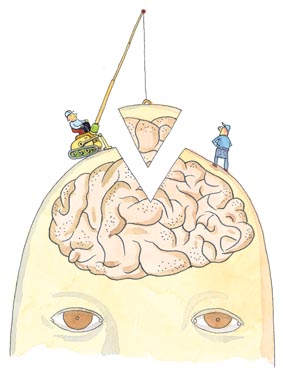
Dudai's team carried out the study with rats and fish. The rats learned to remember flavors; the fish learned to remember flashes of light. In both instances, the animals were trained to associate the stimuli with conflicting memories (the light, for instance, would signal danger only some of the time). In both species, researchers showed that the dominant memory was the only one that could be erased by administering an appropriate drug within a few minutes of recall.
Studies on humans have not yet been conducted, but Dudai points out that the closer we get to the basic principles of memory, the more similarities exist among animals, including humans. Thus drugs found to be effective in eliminating memories in animals may work in humans as well, offering a much awaited piece of good news to trauma sufferers.
DEFINING MEMORY
Dudai's recently published book, Memory from A to Z, provides a unique and valuable introduction to the field of memory for students and researchers alike. It consists of over 130 entries, bound within a coherent conceptual framework. Each entry starts with a definition, or set of definitions, followed by an in-depth and provocative discussion of the origin, meaning, usage and applicability of ideas and problems central to the science of memory and scientific culture at large. The entries provide a versatile tool kit as a source of definitions, information and further reading, as well as a trigger for contemplation, discussion and experimentation. The book can serve as a useful aid to study, teaching and debates, as well as a trigger for future experimentation.
Prof. Dudai's research was supported over the years by the Abe and Kathryn Selsky Foundation; the Nella and Leon Benoziyo Center for Neurosciences; the Lester Crown Brain Research Fund; the Abramson Family Brain Research Program; the Carl and Michaela Einhorn-Dominic Brain Research Institute; and the Murray H. & Meyer Grodetsky Center for Research of Higher Brain Functions. He is the incumbent of the Sara and Michael Sela Professorial Chair of Neurobiology.
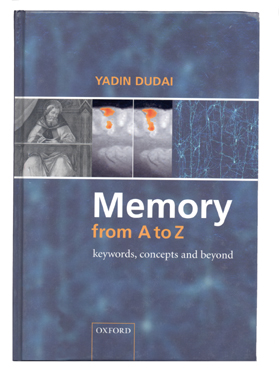
Mopping Up Spills
An enzyme in the blood might be used to prevent brain damage