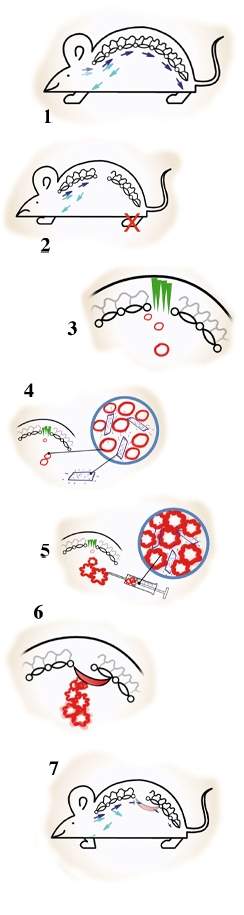
1. Nerve signals from the brain go down the spine to the front and hind limbs
2. Damage to the spine interrupts the signals to the hind legs, causing their paralysis
3. Immune system cells (red) try to heal the damage, but they are blocked by a protective mechanism of the central nervous system (green )
4. A segment of the peripheral nerve (purple) is incubated with the immune cells and "activates" them
5. "Activated" immune cells are returned to the damaged site
6. They manage to overcome the protecitve mechanism an partially heal the damage
7. As a result, the paralysis is partially overcome
Weizmann Institute scientists have managed to partially heal the damaged spinal cords of laboratory animals, according to a study reported in the July issue of Nature Medicine. A team led by Prof. Michal Schwartz of the Neurobiology Department used an innovative treatment which allowed rats to regain partial movement in their hind legs that had been paralyzed by damage to the spine.
"The results of our experiments are promising," says Prof. Schwartz. "However, for the moment, they have been achieved only in rats. A lot of additional research still needs to be done before the new treatment is available to humans."
It has long been known that lower animals, such as fish, can repair damaged fibers in the central nervous system the spinal cord and the brain and restore lost function. In contrast, mammals, including humans, can only repair injuries to the peripheral nerves, while injuries to the brain or spine leave them permanently paralyzed or otherwise handicapped.
The new approach is based on Schwartz's theory which states that the loss of this reparability occurred in the course of evolution, and is due to a unique relationship between the central nervous and immune systems. More specifically, Schwartz believes this loss was probably dictated by the need to protect the mammalian brain from the effects of the immune system. While immune cells normally help to heal damaged tissue, their access to the brain would disrupt the complex and dynamic neural networks that build up during an individual's lifetime.
Generally, when tissue damage occurs, immune cells known as macrophages swarm to the injured site where they remove damaged cells and release substances that promote healing. The central nervous system of mammals is different in this regard: When damaged, it is not effectively assisted by the immune system.
Schwartz's team discovered that this is because the mammalian central nervous system has a mechanism that suppresses the macrophages. As a result, macrophages are recruited to central nervous system injuries at a lower rate, and those that are recruited fail to become optimally "activated" and effective.
These findings led to a series of experiments with rats in the course of which the researchers managed to overcome the limited ability of the damaged central nervous system to recruit and activate the macrophages. They isolated macrophages and incubated them in a test tube in the presence of a damaged peripheral nerve. The macrophages, which received the distress signals of the damaged peripheral nerve, became activated.
At this stage, the researchers returned the activated macrophages to the damaged site in the central nervous system of the paralyzed rat. The transplanted macrophages created a growth-inducing environment around the damaged tissue. As a result of the treatment, the rats were able to regain parti al motor activity in their previously paralyzed legs. They were able to move their hind legs and several animals were even able to place their weight upon them.
A major innovative aspect of such treatment lies in promoting the animal's own self-repair mechanism. In fact, the new treatment offers the option of using the animal's own cells for this purpose.
Further research is necessary to see if this approach will work in higher animals, such as humans
Yeda Research & Development Co. Ltd., the Weizmann Institute's technology transfer arm, has submitted patent applications for the new treatment. In order to promote this research and develop it furt her for possible clinical use, Yeda has entered into a licensing agreement with Proneuron Biotechnology Ltd., a start-up company located in the Kiryat Weizmann Industrial Park adjacent to the Institute.
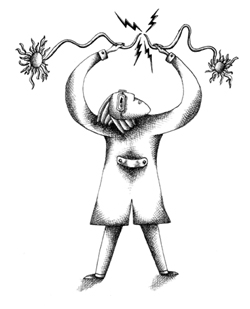
Brain Microprocessors in Action
Brain researchers aim to map nerve cell clusters in action, "conversing" with their peers, processing sensory information, or performing cognitive functions. Each cluster, containing thousands of nerve cells performing a given task, is called a cortical column. The ability to obtain an exact mapping of these columns, which serve as the brain's "microprocessors," is critical to understanding sensory perception and higher cognitive functions. Yet until recently, explorations of the human brain have had to rely on indirect methods with limited accuracy, such as positron emission tomography (PET) and functional magnetic resonance imaging (f-MRI). While these techniques can be used to map an active area at an accuracy of 2-7 mm, mapping brain microprocessors requires an accuracy of 0.5 mm.
In the past 15 years, Prof. Amiram Grinvald of the Weizmann Institute's Neurobiology Department has developed optical imaging - a brain-mapping approach that tracks color changes in the blood ferrying oxygen to active microprocessors. Using this technology, Grinvald was able to identify the exact time and place in which nerve cells consume oxygen from the microcirculation system. The high resolution permitted detailed mapping of individual cortical columns.
Optical imaging laid the foundation for developing functional MRI by Seiji Ogawa and colleagues at AT&T. Initially scientists had hoped that using f-MRI would enable brain mapping with the same accuracy as that obtained by optical imaging. Indeed, both methods detect a considerable "delayed activity peak" that appears roughly six seconds after the onset of electrical activity. Yet the f-MRI systems could not detect the initial dip," a negative signal that appears earlier, which is clearly visible with optical imaging.
This is where things stood until recently, when Grinvald and colleagues published a paper in Science, suggesting how to enhance f-MRI resolution. Now, a team of researchers from Minnesota University has adopted this recipe and found the missing initial dip using high strength magnetic field f-MRI. The marked improvement in f-MRI should assist attempts to probe human cognition and perception. "f-MRI is far more suitable for non-invasive human brain research and clinical applications than optical imaging or PET," says Grinvald. "It may be used to explore the same brain for many years, enabling researchers to track and map memory traces, aging processes, or functional recovery from trauma or stroke."
And the Minnesota team has already collected the first rewards - the first exact mapping of orientation columns, microprocessors responsible for shape perception in the visual cortex.
Prof. Amiram Grinvald holds the Helen and Norman Asher Professorial Chair in Brain Research. His research is supported by the Horace W.Goldsmith Foundation, New York, Murray Meyer Brodetsky Center of Higher Brain Functions, Mrs. Margaret M. Enoch of New York, the Simon and Marie Jaglom Foundation, New York, and the Carl and Michaela Einhorn-Dominic Brain Research Institute, France.