Institute Professor Ephraim Katzir, the fourth president of the State of Israel, an internationally esteemed Israeli scientist and one of the founders of the Weizmann Institute of Science, passed away on Saturday, May 30, 2009, at his home in the Weizmann Institute. He was 93.
Ephraim Katzir was born in Kiev, Ukraine, in 1916. His parents, Yehuda and Tzila Katchalski, brought him to the Land of Israel in 1922. After finishing high school in Jerusalem, he chose to study botany, zoology and bacteriology at the Hebrew University of Jerusalem, though he eventually focused on biochemistry and organic chemistry. In 1941, he completed his doctoral degree with research in simple synthetic polymers of amino acids and continued his studies at the Polytechnic Institute of Brooklyn, Columbia University and Harvard University.
While studying in Jerusalem, Katzir was active in the Hagana and advanced to the rank of field corps company commander. In 1948, after returning from the US, he became involved in military research and development in the Israel army’s Science Corps, “Hemed,” founded at the start of the 1948 War of Independence, and for a time commanded it as a lieutenant colonel.
At the close of the War of Independence, together with his scientist brother, Aharon, Ephraim Katzir joined the faculty of the Weizmann Institute of Science where he established and headed the Biophysics Department. Aharon served as head of the Polymer Department until his murder in a terrorist attack at the Lod airport in 1972.
Over the course of his career, Ephraim Katzir researched synthetic models to investigate the principles of polymer structure and function. His pioneering studies contributed to the deciphering of the genetic code, to the development of synthetic antigens, and to the clarification of the various steps in immune reactions. An understanding of the properties of his models (“poly-amino acids”) aided, among other things, in the development of the multiple sclerosis drug Copaxone® at the Weizmann Institute.
Another of Katzir’s significant successes was the development, in the 1960s, of a method for binding enzymes to various substrates. The method is used today as an important tool in the drug and food industries.
Parallel to his scientific research, Katzir was also active over the years in the social and educational sides of science. He headed a governmental committee that set national science policy, raised generations of new scientists, translated important scientific material into Hebrew and helped to establish a Hebrew-language popular science magazine. He served as the head scientist of the Ministry of Defense and chaired the Society for the Advancement of Science in Israel, the Israeli Biochemical Society, the National Council for Research and Development and the Council for the Advancement of Science Education. In addition, he headed the National Biotechnology Council.
In 1973, Katzir was chosen to be president of the State of Israel (its fourth president), a position he held until 1978. (With his appointment to the presidency, he changed his name from Katchalski to Katzir.) In his term as president, he put special emphasis on societal problems and education and regularly went out of his way to become acquainted with all the different groups making up Israel’s population.
Returning to his scientific career, Katzir’s research took off in new directions. He headed a team that won an international competition for computerized modeling of proteins and took part in a multidisciplinary scientific team that discovered key aspects of the effect of snake venom on the human body. He authored hundreds of scientific articles and served on the editorial and advisory boards of many scientific journals. In honor of his 60th, 70th and 80th birthdays, international scientific symposia were held in Rehovot and Jerusalem. On his 90th birthday, an international scientific conference was held at the Weizmann Institute.
Prof. Katzir was a member of the Israel Academy of Sciences and Humanities and of numerous other learned bodies in Israel and abroad, including the Royal Institution of Great Britain, the Royal Society of London, the National Academy of Sciences of the United States, the Academie des Sciences in France, the Scientific Academy of Argentina and the World Academy of Art and Science. He was a visiting professor at Harvard University, Rockefeller University, the University of California at Los Angeles and Battelle Seattle Research Center.
In addition, Katzir was awarded the Rothschild and Israel Prizes in Natural Sciences, the Weizmann Prize, the Linderstrom Land Gold Medal, the Hans Krebs Medal, the Tchernikovsky Prize for scientific translation, the Alpha Omega Achievement Medal and the Engineering Foundation’s International Award in Enzyme Engineering. He was the first recipient of the Japan Prize and was appointed to France’s Order of the Legion of Honor. He received honorary doctorates from more than a dozen institutions of higher learning in Israel and around the world, including Harvard University, Northwestern University, McGill University, the University of Oxford and the Technion – Israel Institute of Technology.
The official memorial ceremony, held in the Weizmann Institute’s Memorial Plaza, was attended by the president of the state, Shimon Peres, Prime Minister Benjamin Netanyahu and Minister of Defense Ehud Barak, as well as many government ministers, Knesset members, educators, scientists and members of the public.
President Shimon Peres said in his memory: “I have never met another man with such renown and talents as Ephraim, who had so much personal humility combined with such public boldness; boldness in everything related to science, modesty in everything related to the man. He founded the IDF Science Corps (Hemed). When I was director of the Ministry of Defense, the cooperation between us was both full and stimulating. He always created an atmosphere around him of quiet faith.
“From the state’s beginning, Ben-Gurion wanted the president to be a man of science. After Aharon’s murder, then Prime Minister Golda Meir had a heart-to-heart talk with Ephraim and he agreed to take on the mantle of the presidency. Ephraim had a hard time refusing the request, but it was just as hard for him to tear himself away from his lab. He used his scientific savvy in the role of president, working to unite the people, reduce inequality, prevent the polarization of the society and raise its moral standards, as well as to raise the standard of science.”
Prime Minister Benjamin Netanyahu said in his memory: “There are few people whose humility and common touch become greater as they rise in the world. Not many are the people who, even as they ascend to the summit, continue to act with everyday humanity. Ephraim Katzir was such a man. Even when he was president, he was never high and mighty; even when he led groundbreaking research, he never lost sight of the path beneath his feet; even when his name became known around the world, his love for this country remained steadfast and deep. A gracious man, ethical, deep, inquisitive and a lover of his fellow man. Ephraim Katzir was nobly stoic in the face of the loss of his beloved brother, Aharon, who was murdered at the hands of terrorists.
“His whole life, Ephraim Katzir used his rare abilities to contribute to his people and his country. He always made sure that the mission was the main point and not himself – the instrument of the mission.
“This attitude of quiet, modest contribution noticeably accompanied him even when he was chosen to be the fourth president of the State of Israel. History arranged a term for him that began with the Yom Kippur War and ended with peace negotiations with the largest Arab state – Egypt. Yet in this period, President Katzir managed to devote a good part of his time to issues of society and education, higher education and welfare, and he initiated many important projects and good works. And he was always searching for ways to connect youth with science.”
The president of the Weizmann Institute of Science, Prof. Daniel Zajfman, said in his memory: “Ephraim was a brave scientist, who followed his curiosity to the boundaries of human knowledge. In the days when it was hard to imagine a connection between physics and the life sciences, Ephraim founded the Department of Biophysics at the Weizmann Institute of Science. In the days when the State of Israel was having difficulty feeding its citizens, Ephraim looked into the future and decided to research the structure of proteins and their function in the human body.
“But Ephraim didn’t shut himself up in the lab. He saw science as an integral part of human culture and he believed that scientists should lead human society to a better future. Faithful to this belief, he took an extremely active role in explaining science to the general public and he headed the Council for the Advancement of Science Education.
“In Ephraim’s life, Zionism and science were interwoven. It’s not accidental that this ceremony is taking place not far from the burial place of the first president of the State of Israel and of the Weizmann Institute of Science, Chaim Weizmann. It’s no accident that two Weizmann Institute scientists have served as president of the state: Ephraim Katzir and Chaim Weizmann. Both saw Zionism and science as two sides of a whole that is greater than its parts; both believed in the expansion of human knowledge as a means to improve the quality of life for all. Not only were they both right, they proved the justice of their claim.”
Open Questions
In one of Prof. Ephraim Katzir’s last public appearances, he spoke to a group of young people who were at the Weizmann Institute to hear a science lecture in the Katzir-Makineni Lecture Series.
He said: “As someone who has been involved for years in researching life processes, I believe that young people have the originality of thought and the courage to work in any field they choose to engage in.
“When I started studying at the Hebrew University of Jerusalem, I thought I would be a mathematician. I studied mathematics for a whole year and I got great satisfaction from it because there’s a philosophy to mathematics and pure reason. Afterward, I thought that physics was a good subject and I founded the Biophysics Department. In this field, as well, I enjoyed myself, and I saw that a foundation in mathematics is exceptionally beneficial. When I reached the ripe old age of 40, I decided that the life sciences were interesting – and it is, really, a wonderful field. Thus, if any of you choose to go into mathematics and also want to conduct research, I can promise you one thing: There’s outstanding satisfaction in scientific research and there are many more questions waiting for you to discover and investigate. For example: How does one part of the body affect another part? Why do I think in my head that this place is nice? Why, when I’m hungry, does my stomach suddenly start to tell me I’m hungry? A lot of questions are waiting for you to come and answer them and share your knowledge with everyone.”
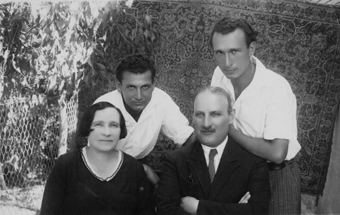
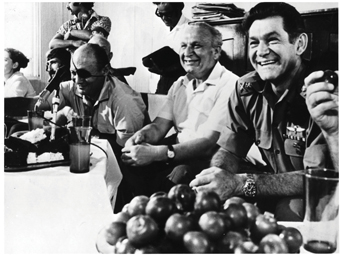
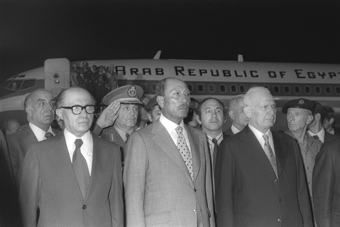
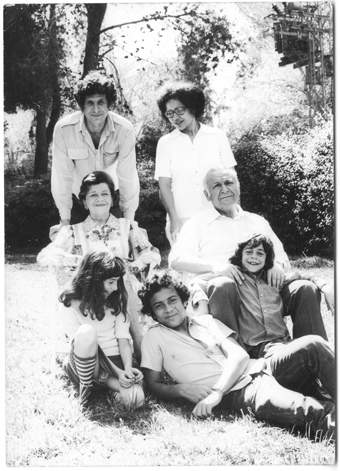
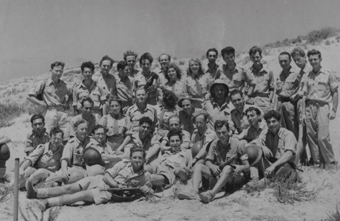
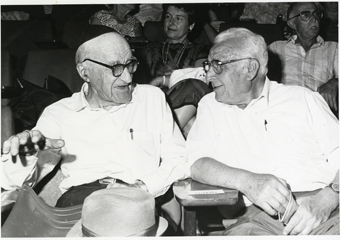
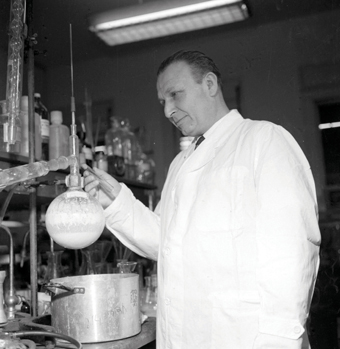
The Math of Distortion
One of the more basic questions is: In a group of objects, how does one decide whether any two are the same or different? This is not a trivial question, even for humans: To the untrained eye, for example, a pile of old animal bones may be a collection of similar objects, but a trained morphologist or paleontologist will be able to sort them into different species. That ability often comes with years of practice and, just as the average person can tell a pear from an apple without much thought, the expert can distinguish between bones without consciously tracing each step leading up to a particular identification. How, then, does one translate this type of thinking, which is at least partly unconscious, to a mathematical computer algorithm?
Lipman and his colleagues developed an algorithm for comparing and classifying anatomical surfaces such as bones and teeth by analyzing plausible deformations among their three-dimensional models. While a human working from a subjective point of view might look for identifying “landmarks” – for example a specific type of recognizable ridge or protrusion – the computer would take a different track: matching the surfaces globally while minimizing the amount of distortion in the match. A human often works from the bottom up, looking for local cues and then putting them together to arrive at a conclusion. In contrast, the algorithm is designed to consider the collective surfaces as a geometric whole and match them in a top-down manner. Lipman then gave his algorithm the ultimate test – pitting its bone and tooth identification against that of expert morphologists. In all the tests, the computer performed nearly as well as the trained morphologists. That means, he says, that non-experts could use the algorithm to quickly obtain accurate species identification from bones and teeth. In the future, further biological information might be more easily extracted from shape.
Dr. Yaron Lipman's research is supported by the Friends of Weizmann Institute in memory of Richard Kronstein