- Into the twilight zone
- Electrons go ballistic
- World record-holders in the electron race
- Chaos in quantum mechanics
- Conspicuous by their absence
Into the twilight zone
Weizmann Institute scientists are among the world's leaders in researching mesoscopic physics. This is the phenomenon occurring in the "twilight zone" between the macroscopic world (which is visible to the eye and where Newtonian physics reigns clearly), and the microscopic world of individual atoms, governed by quantum theory (which states that subatomic particles of matter may appear and behave as either particles or waves).
The Institute's scientists made a significant contribution to understanding the process by which a particle in the quantum state loses its wave properties and begins to behave solely as a particle, and conversely, when particles of matter begin to exhibit wave properties. These properties should cause the electrons to move (under the influence of magnetic fields) in circular currents that never diminish, called persistent currents. This phenomenon occurs in superconductors and, to the surprise of scientists, was recently observed at various laboratories around the world in ordinary conductors.
Another contribution of Weizmann scientists was to more clearly define the limitations of measurement, for example, in cases where researchers wish to note the passage of a single, solitary electron at a specific location. They also contributed to the understanding of the nonlocal influences of waves.
Research in mesoscopic physics may facilitate the development of a new type of miniature electronic device governed mainly by the laws of quantum theory. Measurement instruments based on these devices will allow, for example, the electrical activity of the heart to be measured without invasive procedures or even touching the patient's skin. Future quantum electronics may also lead to the development of supercomputers the size of a pinhead and perhaps the development of minuscule robots.
At the Weizmann Institute's Center for Submicron Research, the only one of its kind in Israel and one of very few in the world, scientists have developed a device that focuses on the wave rather than the particle-like behavior of the electrons. The researchers forced these particle-waves to interfere with each other destructively and constructively, which gave an insight into their wave properties and how the minuscule devices affect the propagation of electrons. Afterwards, the researchers developed a system to measure the rate of passage of these particle-waves, providing knowledge about whether they pass one after another, in a regular periodic pattern in an ordered fashion, or randomly, in a way that cannot be predicted (causing quantum noise). In addition, using precision optics, the researchers examined devices with a relatively small number of electrons including circumstances where the electron's mobility is impaired. The light emitted from these special devices had a significantly different wavelength from that emitted from standard conductors.
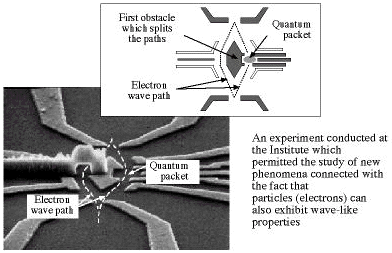
Electrons go ballistic
Weizmann Institute scientists are gradually diminishing the surrounds of electrons moving in electric circuits. When these surroundings are sufficiently small, the electrons move through matter without colliding with other particles. They are then called ballistic electrons because, in addition to their wave properties, they move much faster than regular electrons in today's electronic circuits. Ballistic electrons were known in theory, but the Institute's scientists (working in the United States) were the first to actually discover them.
World record-holders in the electron race
The speed and efficiency of electronic processors depends partly on the time taken by electrons to move through the material from which the processor is made. One way of cutting this time is to make the processor as small as possible, thereby shortening the distance the electrons must travel. Another way is to increase the speed of the electrons.
The speed that electrons can move through a material is governed partly by its purity. The more impurities there are, the more the electrons will collide with foreign particles, which slows them down. The purer the material, the greater the distance covered by the electrons without colliding with other matter.
In the last few years, scientists at advanced research laboratories throughout the world have invested great effort in producing the purest possible semiconductor materials, with the aim of allowing electrons to move within them at increasingly greater speeds. Until recently, the world record in this field was held by scientists at Bell Labs of AT&T in New Jersey, United States. The record velocity was 11.7 million centimeters per second (117 kilometers per second or 73 miles per second) in an electric field of one volt per centimeter.
However, Weizmann Institute scientists developed layers of the world's purest semiconductor (gallium arsenide), which enables electrons moving within it to travel further than ever before without colliding with other particles. The breakthrough enabled the Institute to overtake Bell Labs with a new world record. The gallium arsenide was maintained at a temperature of 0.1 Kelvin (only one-tenth of one degree above absolute zero), allowing electrons to move within it at a speed of 14.4 million centimeters per second (144 kilometers per second, or 89.5 miles per second, equal to 518,400 kilometers per hour or 322,100 miles per hour) in an electric field of one volt per centimeter (2.6 volts per inch).
This pure material was produced in a unique vacuum system installed and enhanced in the Center for Submicron Research at the Weizmann Institute. This system holds the world record for emptiness; it produces the lowest pressure in the world: 10-16 atmospheres.
Gallium arsenide allows the production of electronic devices that are faster and more efficient than silicon ones, as well as light-emitting devices, which is not possible with silicon. For example, it forms the main component of cellular telephones and the laser element in compact disc players. Various studies on gallium arsenide are being conducted at the Center as part of the global race to develop faster and more efficient electronic processors to form the basis for tomorrow's telecommunications and optics industries.
Chaos in quantum mechanics
It has recently become apparent that microscopic systems such as atoms, molecules and submicron electronic components are affected by the chaos phenomenon. This is puzzling because chaotic behavior is usually a property of macroscopic systems. Weizmann Institute scientists are investigating the way chaos is expressed in the quantum world. One of their findings was that "quantum chaos" induces behavior in quantum dynamics which is usually associated with random systems. These studies may be applied to the construction of submicron electronic components and advanced radar systems.
Conspicuous by their absence
Electrons in semiconductor materials skip to a higher energy level when they are illuminated (a phenomenon known as optical excitation). In doing so, they leave behind an electron shortage, nicknamed a "hole." In many senses this hole acts and behaves like a material entity: for example, since the hole is the opposite of the negatively-charged electron, it carries a positive electrical charge. And, like all other subatomic particles, the hole has its own rotational property, known as spin.
In certain circumstances, the electron that skipped to a higher energy level might move around the hole it left behind. This hole, with a positive charge, acts like the nucleus for an atom-like particle called an exciton. Excitons were first observed in clusters of three-dimensional matter at very low temperatures (nearing absolute zero). It has since been clarified that in very thin (two-dimensional) layers of material, excitons may be observed even at room temperature. The electrons in these excitons can only move in one plane.
When one electron moves around the hole, an exciton equivalent to a neutral hydrogen atom is created. Weizmann Institute scientists discovered that in layers of two-dimensional matter, an additional electron (found in semiconductor material) may join the exciton, giving it a negative electrical charge and creating the equivalent of a hydrogen ion.
Excitons are an important model for research because they enable scientists to examine properties and characteristics that are difficult or impossible to test in atoms of regular matter. In addition, controlling exciton properties, such as the absorption and emission of light, may lead to the development and manufacture of new communications and electrooptical devices.